Metabolic changes of human induced pluripotent stem cell-derived cardiomyocytes and teratomas after transplantation
- PMID: 39569381
- PMCID: PMC11576393
- DOI: 10.1016/j.isci.2024.111234
Metabolic changes of human induced pluripotent stem cell-derived cardiomyocytes and teratomas after transplantation
Abstract
Cardiac regenerative therapy using human induced pluripotent stem cell-derived cardiomyocytes (hiPSC-CMs) has been applied in clinical settings. Herein, we aimed to investigate the in vivo metabolic profiles of hiPSC-CM grafts. RNA sequencing and imaging mass spectrometry were performed in the present study, which revealed that hiPSC-CM grafts matured metabolically over time after transplantation. Glycolysis, which was active in the hiPSC-CM grafts immediately after transplantation, shifted to fatty acid oxidation. Additionally, we examined the metabolic profile of teratomas that may form when non-CMs, including undifferentiated human induced pluripotent stem cells (hiPSCs), remain in transplanted cells. The upregulated gene expression of amino acid transporters and the high accumulation of amino acids, such as methionine and aromatic amino acids, were observed in the teratomas. We show that subcutaneous teratomas derived from undifferentiated hiPSCs can be detected in vivo using positron emission tomography with [18F]fluorophenylalanine ([18F]fPhe). These results provided insights into the clinical application of cardiac regenerative therapy.
Keywords: cardiovascular medicine; cell biology.
© 2024 The Author(s).
Conflict of interest statement
K.F. is the co-founder and CEO of Heartseed Inc. S.Tohyama is an advisor from Heartseed Inc. S. Tohyama, H.K., J.F., and K.F. own equity in Heartseed Inc.
Figures
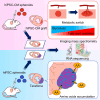
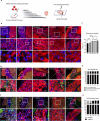
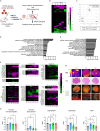
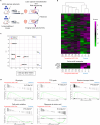
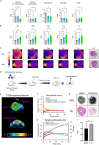
Similar articles
-
Mature human induced pluripotent stem cell-derived cardiomyocytes promote angiogenesis through alpha-B crystallin.Stem Cell Res Ther. 2023 Sep 7;14(1):240. doi: 10.1186/s13287-023-03468-4. Stem Cell Res Ther. 2023. PMID: 37679796 Free PMC article.
-
Production of functional cardiomyocytes and cardiac tissue from human induced pluripotent stem cells for regenerative therapy.J Mol Cell Cardiol. 2022 Mar;164:83-91. doi: 10.1016/j.yjmcc.2021.11.008. Epub 2021 Nov 22. J Mol Cell Cardiol. 2022. PMID: 34822838 Review.
-
Development of appropriate fatty acid formulations to raise the contractility of constructed myocardial tissues.Regen Ther. 2022 Sep 29;21:413-423. doi: 10.1016/j.reth.2022.09.006. eCollection 2022 Dec. Regen Ther. 2022. PMID: 36248630 Free PMC article.
-
Bioorthogonal non-canonical amino acid tagging to track transplanted human induced pluripotent stem cell-specific proteome.Stem Cell Res Ther. 2024 Jun 26;15(1):186. doi: 10.1186/s13287-024-03792-3. Stem Cell Res Ther. 2024. PMID: 38926849 Free PMC article.
-
Unlocking the Pragmatic Potential of Regenerative Therapies in Heart Failure with Next-Generation Treatments.Biomedicines. 2023 Mar 15;11(3):915. doi: 10.3390/biomedicines11030915. Biomedicines. 2023. PMID: 36979894 Free PMC article. Review.
References
-
- Tsao C.W., Aday A.W., Almarzooq Z.I., Alonso A., Beaton A.Z., Bittencourt M.S., Boehme A.K., Buxton A.E., Carson A.P., Commodore-Mensah Y., et al. Heart disease and stroke statistics-2022 update: A report from the American Heart Association. Circulation. 2022;145:e153–e639. doi: 10.1161/cir.0000000000001052. - DOI - PubMed
-
- Tohyama S., Hattori F., Sano M., Hishiki T., Nagahata Y., Matsuura T., Hashimoto H., Suzuki T., Yamashita H., Satoh Y., et al. Distinct metabolic flow enables large-scale purification of mouse and human pluripotent stem cell-derived cardiomyocytes. Cell Stem Cell. 2013;12:127–137. doi: 10.1016/j.stem.2012.09.013. - DOI - PubMed
LinkOut - more resources
Full Text Sources