A non-conducting role of the Cav1.4 Ca2+ channel drives homeostatic plasticity at the cone photoreceptor synapse
- PMID: 39531384
- PMCID: PMC11556788
- DOI: 10.7554/eLife.94908
A non-conducting role of the Cav1.4 Ca2+ channel drives homeostatic plasticity at the cone photoreceptor synapse
Abstract
In congenital stationary night blindness, type 2 (CSNB2)-a disorder involving the Cav1.4 (L-type) Ca2+ channel-visual impairment is mild considering that Cav1.4 mediates synaptic release from rod and cone photoreceptors. Here, we addressed this conundrum using a Cav1.4 knockout (KO) mouse and a knock-in (G369i KI) mouse expressing a non-conducting Cav1.4. Surprisingly, Cav3 (T-type) Ca2+ currents were detected in cones of G369i KI mice and Cav1.4 KO mice but not in cones of wild-type mouse, ground squirrels, and macaque retina. Whereas Cav1.4 KO mice are blind, G369i KI mice exhibit normal photopic (i.e. cone-mediated) visual behavior. Cone synapses, which fail to form in Cav1.4 KO mice, are present, albeit enlarged, and with some errors in postsynaptic wiring in G369i KI mice. While Cav1.4 KO mice lack evidence of cone synaptic responses, electrophysiological recordings in G369i KI mice revealed nominal transmission from cones to horizontal cells and bipolar cells. In CSNB2, we propose that Cav3 channels maintain cone synaptic output provided that the nonconducting role of Cav1.4 in cone synaptogenesis remains intact. Our findings reveal an unexpected form of homeostatic plasticity that relies on a non-canonical role of an ion channel.
Keywords: calcium; ictidomys tridecemlineatus; mouse; neuroscience; photoreceptor; retina; rhesus macaque; synapse.
© 2024, Maddox, Ordemann et al.
Conflict of interest statement
JM, GO, Jd, AH, CG, SW, KR, DF, NS, DM, BZ, SD, MH, AL No competing interests declared
Figures
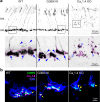
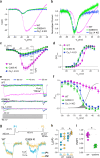
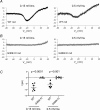
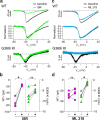
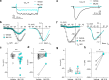
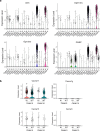
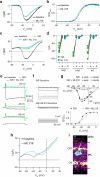
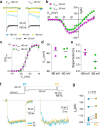
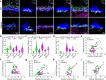
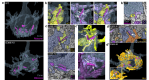
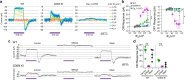
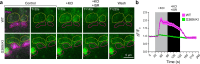
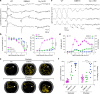
Update of
-
A non-conducting role of the Cav1.4 Ca2+ channel drives homeostatic plasticity at the cone photoreceptor synapse.bioRxiv [Preprint]. 2024 Aug 6:2023.12.05.570129. doi: 10.1101/2023.12.05.570129. bioRxiv. 2024. Update in: Elife. 2024 Nov 12;13:RP94908. doi: 10.7554/eLife.94908. PMID: 38106079 Free PMC article. Updated. Preprint.
Similar articles
-
A non-conducting role of the Cav1.4 Ca2+ channel drives homeostatic plasticity at the cone photoreceptor synapse.bioRxiv [Preprint]. 2024 Aug 6:2023.12.05.570129. doi: 10.1101/2023.12.05.570129. bioRxiv. 2024. Update in: Elife. 2024 Nov 12;13:RP94908. doi: 10.7554/eLife.94908. PMID: 38106079 Free PMC article. Updated. Preprint.
-
Dysregulation of Ca(v)1.4 channels disrupts the maturation of photoreceptor synaptic ribbons in congenital stationary night blindness type 2.Channels (Austin). 2013 Nov-Dec;7(6):514-23. doi: 10.4161/chan.26376. Epub 2013 Sep 24. Channels (Austin). 2013. PMID: 24064553 Free PMC article.
-
Cone dystrophy and ectopic synaptogenesis in a Cacna1f loss of function model of congenital stationary night blindness (CSNB2A).Channels (Austin). 2018 Jan 1;12(1):17-33. doi: 10.1080/19336950.2017.1401688. Epub 2018 Jan 2. Channels (Austin). 2018. PMID: 29179637 Free PMC article.
-
Cav1.4 dysfunction and congenital stationary night blindness type 2.Pflugers Arch. 2021 Sep;473(9):1437-1454. doi: 10.1007/s00424-021-02570-x. Epub 2021 Jul 1. Pflugers Arch. 2021. PMID: 34212239 Free PMC article. Review.
-
The role of voltage-gated ion channels in visual function and disease in mammalian photoreceptors.Pflugers Arch. 2021 Sep;473(9):1455-1468. doi: 10.1007/s00424-021-02595-2. Epub 2021 Jul 13. Pflugers Arch. 2021. PMID: 34255151 Review.
References
-
- Alpadi K, Magupalli VG, Käppel S, Köblitz L, Schwarz K, Seigel GM, Sung C-H, Schmitz F. RIBEYE recruits Munc119, a mammalian ortholog of the Caenorhabditis elegans protein unc119, to synaptic ribbons of photoreceptor synapses. The Journal of Biological Chemistry. 2008;283:26461–26467. doi: 10.1074/jbc.M801625200. - DOI - PMC - PubMed
-
- Bech-Hansen NT, Naylor MJ, Maybaum TA, Pearce WG, Koop B, Fishman GA, Mets M, Musarella MA, Boycott KM. Loss-of-function mutations in a calcium-channel alpha1-subunit gene in Xp11.23 cause incomplete X-linked congenital stationary night blindness. Nature Genetics. 1998;19:264–267. doi: 10.1038/947. - DOI - PubMed
-
- Bimonte-Nelson H, Daniel J, Koebele S. The Maze Book: Theories, Practice, and Protocols for Testing Rodent Cognition. Springer; 2015. - DOI
MeSH terms
Substances
Supplementary concepts
Grants and funding
LinkOut - more resources
Full Text Sources
Research Materials
Miscellaneous