Advances in Microengineered Platforms for Skin Research
- PMID: 39525704
- PMCID: PMC11550131
- DOI: 10.1016/j.xjidi.2024.100315
Advances in Microengineered Platforms for Skin Research
Abstract
The skin plays a critical role in human physiology, acting both as a barrier to environmental insults and as a window to environmental stimuli. Disruption of this homeostasis leads to numerous skin disorders. Human and animal skin differ significantly, limiting the translational potential of animal-based investigations to advance therapeutics to human skin diseases. Hence, there is a critical need for physiologically relevant human skin models to explore novel treatment strategies. Recent advances in microfluidic technologies now allow design and generation of organ-on-chip devices that mimic critical features of tissue architecture. Skin-on-a-chip and microfluidic platforms hold promise as useful models for diverse dermatology applications. Compared with traditional in vitro models, microfluidic platforms offer improved control of fluid flow, which in turn allows precise manipulation of cell and molecular distribution. These properties enable the generation of multilayered in vitro models that mimic human skin structure while simultaneously offering superior control over nutrient and drug distribution. Researchers have used microfluidic platforms for a variety of applications in skin research, including epidermal-dermal cellular crosstalk, cell migration, mechanobiology, microbiome-immune response interactions, vascular biology, and wound healing. In this review, we comprehensively review state-of-the-art microfluidic models for skin research. We discuss the challenges and promise of current skin-on-a-chip technologies and provide a roadmap for future research in this active field.
Keywords: In vitro culture; Microfluidics; Organ-on-a-chip; Skin models.
© 2024 The Authors.
Figures
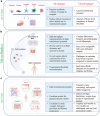
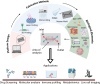
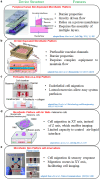
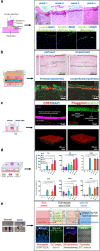
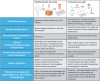
Similar articles
-
Microengineered Organ-on-a-chip Platforms towards Personalized Medicine.Curr Pharm Des. 2018;24(45):5354-5366. doi: 10.2174/1381612825666190222143542. Curr Pharm Des. 2018. PMID: 30799783 Review.
-
Microfluidics-based in vivo mimetic systems for the study of cellular biology.Acc Chem Res. 2014 Apr 15;47(4):1165-73. doi: 10.1021/ar4002608. Epub 2014 Feb 20. Acc Chem Res. 2014. PMID: 24555566 Free PMC article.
-
Current advances in skin-on-a-chip models for drug testing.Microphysiol Syst. 2018 Aug;2:4. doi: 10.21037/mps.2018.08.01. Epub 2018 Aug 30. Microphysiol Syst. 2018. PMID: 33521629 Free PMC article.
-
Evolution of Biochip Technology: A Review from Lab-on-a-Chip to Organ-on-a-Chip.Micromachines (Basel). 2020 Jun 18;11(6):599. doi: 10.3390/mi11060599. Micromachines (Basel). 2020. PMID: 32570945 Free PMC article. Review.
-
Engineering Tissue Barrier Models on Hydrogel Microfluidic Platforms.ACS Appl Mater Interfaces. 2021 Mar 31;13(12):13920-13933. doi: 10.1021/acsami.0c21573. Epub 2021 Mar 19. ACS Appl Mater Interfaces. 2021. PMID: 33739812 Review.
References
-
- Agarwal T., Narayana G.H., Banerjee I. Keratinocytes are mechanoresponsive to the microflow-induced shear stress. Cytoskeleton (Hoboken) 2019;76:209–218. - PubMed
Publication types
Grants and funding
LinkOut - more resources
Full Text Sources