An MIP-Based PFAS Sensor Exploiting Nanolayers on Plastic Optical Fibers for Ultra-Wide and Ultra-Low Detection Ranges-A Case Study of PFAS Detection in River Water
- PMID: 39513844
- PMCID: PMC11547922
- DOI: 10.3390/nano14211764
An MIP-Based PFAS Sensor Exploiting Nanolayers on Plastic Optical Fibers for Ultra-Wide and Ultra-Low Detection Ranges-A Case Study of PFAS Detection in River Water
Abstract
In this work, a novel optical-chemical sensor for the detection of per- and polyfluorinated substances (PFASs) in a real scenario is presented. The proposed sensing approach exploits the multimode characteristics of plastic optical fibers (POFs) to achieve unconventional sensors via surface plasmon resonance (SPR) phenomena. The sensor is realized by the coupling of an SPR-POF platform with a novel chemical chip based on different polymeric nanolayers over the core of a D-shaped POF, one made up of an optical adhesive and one of a molecularly imprinted polymer (MIP) for PFAS. The chemical chip is used to launch the light into the SPR D-shaped POF platform, so the interaction between the analyte and the MIP's sites can be used to modulate the propagated light in the POFs and the SPR phenomena. Selectivity tests and dose-response curves by standard PFOA water solutions were carried out to characterize the detection range sensor response, obtaining a wide PFAS response range, from 1 ppt to 1000 ppt. Then, tests performed on river water samples collected from the Bormida river paved the way for the applicability of the proposed approach to a real scenario.
Keywords: UV-curable optical adhesive; molecularly imprinted polymers (MIPs); per- and polyfluorinated substances (PFASs); perfluorooctanoic acid (PFOA); persistent organic pollutants (POPs); plastic optical fibers (POFs); surface plasmon resonance (SPR).
Conflict of interest statement
The authors declare no conflicts of interest.
Figures
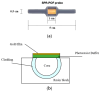
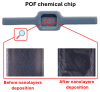
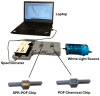
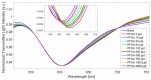
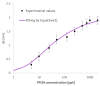
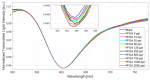
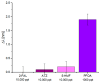
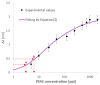
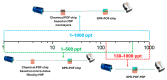
Similar articles
-
Detection of 2-Furaldehyde in Milk by MIP-Based POF Chips Combined with an SPR-POF Sensor.Sensors (Basel). 2022 Oct 28;22(21):8289. doi: 10.3390/s22218289. Sensors (Basel). 2022. PMID: 36365986 Free PMC article.
-
A Simple and Low-Cost Optical Fiber Intensity-Based Configuration for Perfluorinated Compounds in Water Solution.Sensors (Basel). 2018 Sep 8;18(9):3009. doi: 10.3390/s18093009. Sensors (Basel). 2018. PMID: 30205565 Free PMC article.
-
A Molecularly Imprinted Polymer on a Plasmonic Plastic Optical Fiber to Detect Perfluorinated Compounds in Water.Sensors (Basel). 2018 Jun 5;18(6):1836. doi: 10.3390/s18061836. Sensors (Basel). 2018. PMID: 29874860 Free PMC article.
-
A Novel Approach to Realize Plasmonic Sensors via Multimode Optical Waveguides: A Review.Sensors (Basel). 2023 Jun 17;23(12):5662. doi: 10.3390/s23125662. Sensors (Basel). 2023. PMID: 37420827 Free PMC article. Review.
-
Molecularly imprinted polymers for per- and polyfluoroalkyl substances enrichment and detection.Talanta. 2023 Jun 1;258:124434. doi: 10.1016/j.talanta.2023.124434. Epub 2023 Mar 13. Talanta. 2023. PMID: 36940572 Review.
References
-
- Stoiber T., Evans S., Temkin A.M., Andrews D.Q., Naidenko O.V. PFAS in drinking water: An emergent water quality threat. Water Solut. 2020;1:e49.
Grants and funding
LinkOut - more resources
Full Text Sources