Developmental bias as a cause and consequence of adaptive radiation and divergence
- PMID: 39479512
- PMCID: PMC11521891
- DOI: 10.3389/fcell.2024.1453566
Developmental bias as a cause and consequence of adaptive radiation and divergence
Abstract
Efforts to reconcile development and evolution have demonstrated that development is biased, with phenotypic variation being more readily produced in certain directions. However, how this "developmental bias" can influence micro- and macroevolution is poorly understood. In this review, we demonstrate that defining features of adaptive radiations suggest a role for developmental bias in driving adaptive divergence. These features are i) common ancestry of developmental systems; ii) rapid evolution along evolutionary "lines of least resistance;" iii) the subsequent repeated and parallel evolution of ecotypes; and iv) evolutionary change "led" by biased phenotypic plasticity upon exposure to novel environments. Drawing on empirical and theoretical data, we highlight the reciprocal relationship between development and selection as a key driver of evolutionary change, with development biasing what variation is exposed to selection, and selection acting to mold these biases to align with the adaptive landscape. Our central thesis is that developmental biases are both the causes and consequences of adaptive radiation and divergence. We argue throughout that incorporating development and developmental bias into our thinking can help to explain the exaggerated rate and scale of evolutionary processes that characterize adaptive radiations, and that this can be best achieved by using an eco-evo-devo framework incorporating evolutionary biology, development, and ecology. Such a research program would demonstrate that development is not merely a force that imposes constraints on evolution, but rather directs and is directed by evolutionary forces. We round out this review by highlighting key gaps in our understanding and suggest further research programs that can help to resolve these issues.
Keywords: eco-evo-devo; ecotype evolution; evolvability; extended evolutionary synthesis; parallel evolution; phenotypic plasticity; plasticity-led evolution; quantitative genetics.
Copyright © 2024 Stansfield and Parsons.
Conflict of interest statement
The authors declare that the research was conducted in the absence of any commercial or financial relationships that could be construed as a potential conflict of interest.
Figures
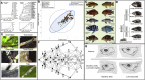
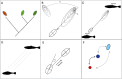
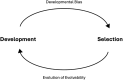
Similar articles
-
A way forward with eco evo devo: an extended theory of resource polymorphism with postglacial fishes as model systems.Biol Rev Camb Philos Soc. 2019 Oct;94(5):1786-1808. doi: 10.1111/brv.12534. Epub 2019 Jun 19. Biol Rev Camb Philos Soc. 2019. PMID: 31215138 Free PMC article. Review.
-
Evolutionary Connectionism: Algorithmic Principles Underlying the Evolution of Biological Organisation in Evo-Devo, Evo-Eco and Evolutionary Transitions.Evol Biol. 2016;43(4):553-581. doi: 10.1007/s11692-015-9358-z. Epub 2015 Dec 8. Evol Biol. 2016. PMID: 27932852 Free PMC article.
-
A mathematical framework for evo-devo dynamics.Theor Popul Biol. 2024 Feb;155:24-50. doi: 10.1016/j.tpb.2023.11.003. Epub 2023 Dec 2. Theor Popul Biol. 2024. PMID: 38043588
-
Rules of teeth development align microevolution with macroevolution in extant and extinct primates.Nat Ecol Evol. 2023 Oct;7(10):1729-1739. doi: 10.1038/s41559-023-02167-w. Epub 2023 Aug 31. Nat Ecol Evol. 2023. PMID: 37652997
-
Developmental Bias and Evolution: A Regulatory Network Perspective.Genetics. 2018 Aug;209(4):949-966. doi: 10.1534/genetics.118.300995. Genetics. 2018. PMID: 30049818 Free PMC article. Review.
References
Publication types
Grants and funding
LinkOut - more resources
Full Text Sources