Helicase protein DDX11 as a novel antiviral factor promoting RIG-I-MAVS-mediated signaling pathway
- PMID: 39470258
- PMCID: PMC11633105
- DOI: 10.1128/mbio.02028-24
Helicase protein DDX11 as a novel antiviral factor promoting RIG-I-MAVS-mediated signaling pathway
Abstract
Type Ι interferon (IFN) production mediated by retinoic acid-inducible gene 1 (RIG-I) and mitochondrial antiviral signaling protein (MAVS) is essential for antiviral innate immune responses. Here, we report the identification of a novel co-sensor for cytosolic nucleic acids: DEAD/H-box helicase 11 (DDX11), a member of the DExD/H (Asp-Glu-x-Asp/His)-box helicase family. Knockdown or knockout of DDX11 attenuated the ability of cells to increase IFN-β, IFN-stimulated gene 56, and C-X-C motif chemokine ligand 10 in response to SeV and poly (I:C) by blocking the activation of TANK-binding kinase 1 and IFN regulatory factor 3. Nucleic acid sensing by DDX11 was independent of the stimulator of IFN genes but was dependent on RIG-I and MAVS. DDX11 regulated RIG-I-MAVS-mediated IFN signaling by specifically interacting with nucleic acid, RIG-I, and MAVS to enhance RIG-I-double-strand RNA and RIG-I-MAVS binding affinity. Overall, our results identified a critical role for DDX11 in the innate immune response and provided molecular insights into the mechanisms by which DDX11 recognized cytosolic nucleic acid and interacted with RIG-Ι and MAVS for potent IFN signaling and antiviral immunity.
Importance: Innate immunity is the first and most rapid host defense against virus infection. Recognition of viral RNA by the retinoic acid-inducible gene 1 (RIG-I)-like receptors (RLRs) initiates innate antiviral immune responses. How the binding of viral RNA to and activation of the RLRs are regulated remains enigmatic. In this study, we identified DEAD/H-box helicase 11 (DDX11) as a positive regulator of the RIG-I-mitochondrial antiviral signaling protein (MAVS)-mediated signaling pathways. Mechanistically, we demonstrated that DDX11 bound to viral RNA, interacted with RIG-I, and promoted their binding to viral RNA. DDX11 also promoted the interaction between RIG-I and MAVS and activation of RIG-I-MAVS signaling. Overall, our results elucidate the role of DDX11 in RIG-I-MAVS-dependent signaling pathways and may shed light on innate immune gene regulation.
Keywords: DDX11; IFN; MAVS; RIG-I.
Conflict of interest statement
The authors declare no conflict of interest.
Figures
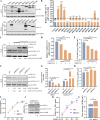
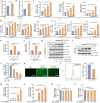
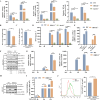
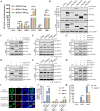
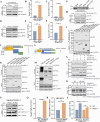
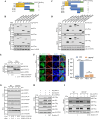
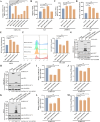
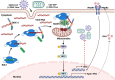
Similar articles
-
Hepatitis C Virus Infection Is Inhibited by a Noncanonical Antiviral Signaling Pathway Targeted by NS3-NS4A.J Virol. 2019 Nov 13;93(23):e00725-19. doi: 10.1128/JVI.00725-19. Print 2019 Dec 1. J Virol. 2019. PMID: 31534039 Free PMC article.
-
Targeting PRMT7-mediated monomethylation of MAVS enhances antiviral innate immune responses and inhibits RNA virus replication.Proc Natl Acad Sci U S A. 2024 Nov 19;121(47):e2408117121. doi: 10.1073/pnas.2408117121. Epub 2024 Nov 15. Proc Natl Acad Sci U S A. 2024. PMID: 39546576 Free PMC article.
-
LRRC59 modulates type I interferon signaling by restraining the SQSTM1/p62-mediated autophagic degradation of pattern recognition receptor DDX58/RIG-I.Autophagy. 2020 Mar;16(3):408-418. doi: 10.1080/15548627.2019.1615303. Epub 2019 May 22. Autophagy. 2020. PMID: 31068071 Free PMC article.
-
Depressing time: Waiting, melancholia, and the psychoanalytic practice of care.In: Kirtsoglou E, Simpson B, editors. The Time of Anthropology: Studies of Contemporary Chronopolitics. Abingdon: Routledge; 2020. Chapter 5. In: Kirtsoglou E, Simpson B, editors. The Time of Anthropology: Studies of Contemporary Chronopolitics. Abingdon: Routledge; 2020. Chapter 5. PMID: 36137063 Free Books & Documents. Review.
-
Impact of residual disease as a prognostic factor for survival in women with advanced epithelial ovarian cancer after primary surgery.Cochrane Database Syst Rev. 2022 Sep 26;9(9):CD015048. doi: 10.1002/14651858.CD015048.pub2. Cochrane Database Syst Rev. 2022. PMID: 36161421 Free PMC article. Review.
References
-
- Kato H, Takeuchi O, Sato S, Yoneyama M, Yamamoto M, Matsui K, Uematsu S, Jung A, Kawai T, Ishii KJ, Yamaguchi O, Otsu K, Tsujimura T, Koh C-S, Reis e Sousa C, Matsuura Y, Fujita T, Akira S. 2006. Differential roles of MDA5 and RIG-I helicases in the recognition of RNA viruses. Nature 441:101–105. doi:10.1038/nature04734 - DOI - PubMed
MeSH terms
Substances
Grants and funding
LinkOut - more resources
Full Text Sources
Miscellaneous