Binding of a single nitric oxide molecule is sufficient to disrupt DNA binding of the nitrosative stress regulator NsrR
- PMID: 39464610
- PMCID: PMC11500311
- DOI: 10.1039/d4sc04618h
Binding of a single nitric oxide molecule is sufficient to disrupt DNA binding of the nitrosative stress regulator NsrR
Abstract
The regulatory protein NsrR, a member of the Rrf2 protein superfamily, plays a major role in the cellular response to nitrosative stress in many benign and pathogenic bacteria. The homodimeric protein binds a [4Fe-4S] cluster in each subunit (termed holo NsrR), and represses transcription of genes primarily involved in NO detoxification. Holo NsrR reacts rapidly with multiple NO molecules per [4Fe-4S] cluster, via a complex reaction, with loss of DNA binding and formation of NsrR-bound iron-nitrosyl species. However, the point at which DNA binding is lost is unknown. Here, we demonstrate using surface plasmon resonance (SPR) and native mass spectrometry (MS) that holo NsrR binds the promoter regions of NsrR-regulated genes with promoter-dependent nanomolar affinity, while hemi-apo NsrR (i.e. one cluster per dimer) binds >10-fold less tightly, and the cluster-free (apo) form not at all. Strikingly, native MS provided detailed information about the reaction of NO with the physiologically relevant form of NsrR, i.e. DNA-bound dimeric NsrR. Reaction with a single NO molecule per NsrR dimer is sufficient to abolish DNA binding. This exquisite sensitivity of DNA binding to NO is consistent with the importance of de-repressing NO detoxification systems at the earliest opportunity to minimise damage due to nitrosative stress. Furthermore, the data show that previously characterised iron-nitrosyls, which form at higher ratios of NO to [4Fe-4S], are not physiologically relevant for regulating the NsrR on/off switch.
This journal is © The Royal Society of Chemistry.
Conflict of interest statement
The authors have no conflicts to declare.
Figures
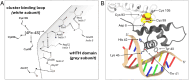
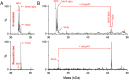
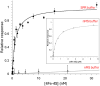
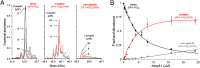
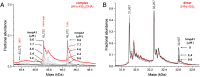
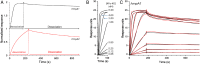
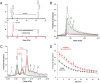
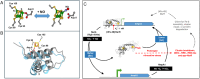
Similar articles
-
NsrR from Streptomyces coelicolor is a nitric oxide-sensing [4Fe-4S] cluster protein with a specialized regulatory function.J Biol Chem. 2015 May 15;290(20):12689-704. doi: 10.1074/jbc.M115.643072. Epub 2015 Mar 14. J Biol Chem. 2015. PMID: 25771538 Free PMC article.
-
Differentiated, Promoter-specific Response of [4Fe-4S] NsrR DNA Binding to Reaction with Nitric Oxide.J Biol Chem. 2016 Apr 15;291(16):8663-72. doi: 10.1074/jbc.M115.693192. Epub 2016 Feb 17. J Biol Chem. 2016. PMID: 26887943 Free PMC article.
-
Transcription Factor NsrR from Bacillus subtilis Senses Nitric Oxide with a 4Fe-4S Cluster (†).Biochemistry. 2008 Dec 9;47(49):13084-92. doi: 10.1021/bi801342x. Biochemistry. 2008. PMID: 19006327 Free PMC article.
-
Iron-sulfur clusters as biological sensors: the chemistry of reactions with molecular oxygen and nitric oxide.Acc Chem Res. 2014 Oct 21;47(10):3196-205. doi: 10.1021/ar5002507. Epub 2014 Sep 29. Acc Chem Res. 2014. PMID: 25262769 Review.
-
Reactions of nitric oxide and oxygen with the regulator of fumarate and nitrate reduction, a global transcriptional regulator, during anaerobic growth of Escherichia coli.Methods Enzymol. 2008;437:191-209. doi: 10.1016/S0076-6879(07)37011-0. Methods Enzymol. 2008. PMID: 18433630 Review.
References
-
- Toledo Jr J. C. Augusto O. Chem. Res. Toxicol. 2012;25:975–989. - PubMed
Grants and funding
LinkOut - more resources
Full Text Sources