YY1 is a transcriptional activator of the mouse LINE-1 Tf subfamily
- PMID: 39460630
- PMCID: PMC11602158
- DOI: 10.1093/nar/gkae949
YY1 is a transcriptional activator of the mouse LINE-1 Tf subfamily
Abstract
Long interspersed element type 1 (LINE-1, L1) is an active autonomous transposable element in human and mouse genomes. L1 transcription is controlled by an internal RNA polymerase II promoter in the 5' untranslated region (5'UTR) of a full-length L1. It has been shown that transcription factor YY1 binds to a conserved sequence at the 5' end of the human L1 5'UTR and primarily dictates where transcription initiates. Putative YY1-binding motifs have been predicted in the 5'UTRs of two distinct mouse L1 subfamilies, Tf and Gf. Using site-directed mutagenesis, in vitro binding and gene knockdown assays, we experimentally tested the role of YY1 in mouse L1 transcription. Our results indicate that Tf, but not Gf subfamily, harbors functional YY1-binding sites in 5'UTR monomers and YY1 functions as a transcriptional activator for the mouse Tf subfamily. Activation of Tf transcription by YY1 during early embryogenesis is also supported by a reanalysis of published zygotic knockdown data. Furthermore, YY1-binding motifs are solely responsible for the synergistic interaction between Tf monomers, consistent with a model wherein distant monomers act as enhancers for mouse L1 transcription. The abundance of YY1-binding sites in Tf elements also raise important implications for gene regulation across the genome.
© The Author(s) 2024. Published by Oxford University Press on behalf of Nucleic Acids Research.
Figures
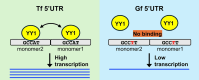
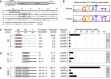
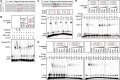
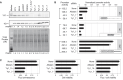
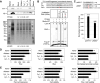
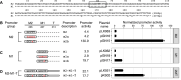
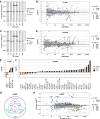
Update of
-
YY1 is a transcriptional activator of mouse LINE-1 Tf subfamily.bioRxiv [Preprint]. 2024 Jan 4:2024.01.03.573552. doi: 10.1101/2024.01.03.573552. bioRxiv. 2024. Update in: Nucleic Acids Res. 2024 Nov 27;52(21):12878-12894. doi: 10.1093/nar/gkae949 PMID: 38260579 Free PMC article. Updated. Preprint.
Similar articles
-
YY1 is a transcriptional activator of mouse LINE-1 Tf subfamily.bioRxiv [Preprint]. 2024 Jan 4:2024.01.03.573552. doi: 10.1101/2024.01.03.573552. bioRxiv. 2024. Update in: Nucleic Acids Res. 2024 Nov 27;52(21):12878-12894. doi: 10.1093/nar/gkae949 PMID: 38260579 Free PMC article. Updated. Preprint.
-
Depressing time: Waiting, melancholia, and the psychoanalytic practice of care.In: Kirtsoglou E, Simpson B, editors. The Time of Anthropology: Studies of Contemporary Chronopolitics. Abingdon: Routledge; 2020. Chapter 5. In: Kirtsoglou E, Simpson B, editors. The Time of Anthropology: Studies of Contemporary Chronopolitics. Abingdon: Routledge; 2020. Chapter 5. PMID: 36137063 Free Books & Documents. Review.
-
Defining the optimum strategy for identifying adults and children with coeliac disease: systematic review and economic modelling.Health Technol Assess. 2022 Oct;26(44):1-310. doi: 10.3310/ZUCE8371. Health Technol Assess. 2022. PMID: 36321689 Free PMC article.
-
Comparison of Two Modern Survival Prediction Tools, SORG-MLA and METSSS, in Patients With Symptomatic Long-bone Metastases Who Underwent Local Treatment With Surgery Followed by Radiotherapy and With Radiotherapy Alone.Clin Orthop Relat Res. 2024 Dec 1;482(12):2193-2208. doi: 10.1097/CORR.0000000000003185. Epub 2024 Jul 23. Clin Orthop Relat Res. 2024. PMID: 39051924
-
Impact of residual disease as a prognostic factor for survival in women with advanced epithelial ovarian cancer after primary surgery.Cochrane Database Syst Rev. 2022 Sep 26;9(9):CD015048. doi: 10.1002/14651858.CD015048.pub2. Cochrane Database Syst Rev. 2022. PMID: 36161421 Free PMC article. Review.
References
-
- Singer M.F. SINEs and LINEs: highly repeated short and long interspersed sequences in mammalian genomes. Cell. 1982; 28:433–434. - PubMed
-
- Smit A.F., Toth G., Riggs A.D., Jurka J.. Ancestral, mammalian-wide subfamilies of LINE-1 repetitive sequences. J. Mol. Biol. 1995; 246:401–417. - PubMed
-
- Saha P.S., An W.. eLS. 2019; (Chichester: John Wiley & Sons, Ltd Ed; 1–10.
MeSH terms
Substances
Grants and funding
LinkOut - more resources
Full Text Sources
Miscellaneous