A plasmonic biosensor pre-diagnostic tool for Familial Mediterranean Fever
- PMID: 39353949
- PMCID: PMC11445562
- DOI: 10.1038/s41467-024-52961-8
A plasmonic biosensor pre-diagnostic tool for Familial Mediterranean Fever
Abstract
Familial Mediterranean Fever (FMF) is an autosomal recessive genetic disorder, primarily observed in populations around the Mediterranean Sea, linked to MEFV gene mutations. These mutations disrupt inflammatory responses, increasing pyrin-protein production. Traditional diagnosis relies on clinical symptoms, family history, acute phase reactants, and excluding similar syndromes with MEFV testing, which is expensive and often inconclusive due to heterozygous mutations. Here, we present a biosensor platform that detects differences in pyrin-protein levels between healthy and affected individuals, offering a cost-effective alternative to genetic testing. Our platform uses gold nanoparticle-based plasmonic chips enhanced with anti-pyrin antibodies, achieving a detection limit of 0.24 ng/mL with high specificity. The system integrates an optofluidic system and visible light spectroscopy for real-time analysis, with signal stability maintained for up to six months. Our technology will enhance FMF diagnosis accuracy, enabling early treatment initiation and providing a cost-effective alternative to genetic testing, thus improving patient care.
© 2024. The Author(s).
Conflict of interest statement
I.K.A., S.N.T., C.A., E.U., B.M., S.K., B.A., I.Y., T.S., and A.E.C. have a pending patent application for the pre-diagnostic technology presented in this paper. The remaining authors declare no competing interests.
Figures
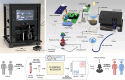
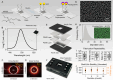
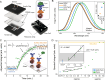
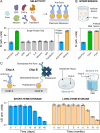
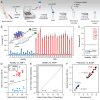
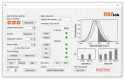
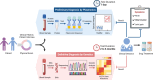
Similar articles
-
Diagnosis of familial Mediterranean fever following the initial presentation of monoarthritis.Int J Rheum Dis. 2018 Mar;21(3):755-760. doi: 10.1111/1756-185X.12906. Epub 2016 Jun 16. Int J Rheum Dis. 2018. PMID: 27310037
-
Familial Mediterranean fever with a single MEFV mutation: where is the second hit?Arthritis Rheum. 2009 Jun;60(6):1851-61. doi: 10.1002/art.24569. Arthritis Rheum. 2009. PMID: 19479870 Free PMC article.
-
R202Q/M694V as novel MEFV gene mutations in chronic periodontitis and familial Mediterranean fever.J Periodontal Res. 2017 Dec;52(6):994-1003. doi: 10.1111/jre.12467. Epub 2017 Jun 7. J Periodontal Res. 2017. PMID: 28590056
-
[Role of genetics in familial Mediterranean fever].Z Rheumatol. 2017 May;76(4):303-312. doi: 10.1007/s00393-017-0265-9. Z Rheumatol. 2017. PMID: 28154935 Review. German.
-
The significance of carrying MEFV variants in symptomatic and asymptomatic individuals.Clin Genet. 2024 Sep;106(3):217-223. doi: 10.1111/cge.14566. Epub 2024 May 31. Clin Genet. 2024. PMID: 38818540 Review.
References
-
- Portincasa, P., Scaccianoce, G. & Palasciano, G. Familial Mediterranean fever: a fascinating model of inherited autoinflammatory disorder. Eur. J. Clin. Invest.43, 1314–1327 (2013). - PubMed
-
- Ben-Chetrit, E. & Touitou, I. Familial Mediterranean fever in the world. Arthritis Rheum.61, 1447–1453 (2009). - PubMed
-
- The French FMF Consortium. et al. A candidate gene for familial Mediterranean fever. Nat. Genet.17, 25–31 (1997). - PubMed
-
- Sood, R. et al. Construction of a 1-Mb restriction-mapped cosmid contig containing the candidate region for the familial Mediterranean fever locus (MEFV) on chromosome 16p13.3. Genomics42, 83–95 (1997). - PubMed
MeSH terms
Substances
Grants and funding
LinkOut - more resources
Full Text Sources