Unveiling the Connection: Viral Infections and Genes in dNTP Metabolism
- PMID: 39339888
- PMCID: PMC11437409
- DOI: 10.3390/v16091412
Unveiling the Connection: Viral Infections and Genes in dNTP Metabolism
Abstract
Deoxynucleoside triphosphates (dNTPs) are crucial for the replication and maintenance of genomic information within cells. The balance of the dNTP pool involves several cellular enzymes, including dihydrofolate reductase (DHFR), ribonucleotide reductase (RNR), and SAM and HD domain-containing protein 1 (SAMHD1), among others. DHFR is vital for the de novo synthesis of purines and deoxythymidine monophosphate, which are necessary for DNA synthesis. SAMHD1, a ubiquitously expressed deoxynucleotide triphosphohydrolase, converts dNTPs into deoxynucleosides and inorganic triphosphates. This process counteracts the de novo dNTP synthesis primarily carried out by RNR and cellular deoxynucleoside kinases, which are most active during the S phase of the cell cycle. The intracellular levels of dNTPs can influence various viral infections. This review provides a concise summary of the interactions between different viruses and the genes involved in dNTP metabolism.
Keywords: SAMHD1; deoxynucleoside triphosphates; dihydrofolate reductase; ribonucleotide reductase; viruses.
Conflict of interest statement
The authors declare no conflicts of interest. The funders had no role in the design of the study; in the collection, analyses, or interpretation of data; in the writing of the manuscript; or in the decision to publish the results.
Figures
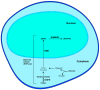
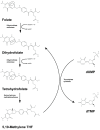
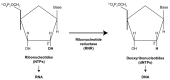
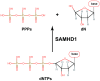
Similar articles
-
SAMHD1 Functions and Human Diseases.Viruses. 2020 Mar 31;12(4):382. doi: 10.3390/v12040382. Viruses. 2020. PMID: 32244340 Free PMC article. Review.
-
The deoxynucleotide triphosphohydrolase SAMHD1 is a major regulator of DNA precursor pools in mammalian cells.Proc Natl Acad Sci U S A. 2013 Aug 27;110(35):14272-7. doi: 10.1073/pnas.1312033110. Epub 2013 Jul 15. Proc Natl Acad Sci U S A. 2013. PMID: 23858451 Free PMC article.
-
p21 Restricts HIV-1 in Monocyte-Derived Dendritic Cells through the Reduction of Deoxynucleoside Triphosphate Biosynthesis and Regulation of SAMHD1 Antiviral Activity.J Virol. 2017 Nov 14;91(23):e01324-17. doi: 10.1128/JVI.01324-17. Print 2017 Dec 1. J Virol. 2017. PMID: 28931685 Free PMC article.
-
The dNTP triphosphohydrolase activity of SAMHD1 persists during S-phase when the enzyme is phosphorylated at T592.Cell Cycle. 2018;17(9):1102-1114. doi: 10.1080/15384101.2018.1480216. Epub 2018 Jul 24. Cell Cycle. 2018. PMID: 30039733 Free PMC article.
-
Involvement of SAMHD1 in dNTP homeostasis and the maintenance of genomic integrity and oncotherapy (Review).Int J Oncol. 2020 Apr;56(4):879-888. doi: 10.3892/ijo.2020.4988. Epub 2020 Feb 14. Int J Oncol. 2020. PMID: 32319570 Review.
Cited by
-
Exploring the Frontiers of Virus-Host Interactions-3rd Edition.Viruses. 2024 Sep 30;16(10):1544. doi: 10.3390/v16101544. Viruses. 2024. PMID: 39459878 Free PMC article.
References
Publication types
MeSH terms
Substances
Grants and funding
LinkOut - more resources
Full Text Sources
Medical
Research Materials
Miscellaneous