Metal-Based Drug-DNA Interactions and Analytical Determination Methods
- PMID: 39339356
- PMCID: PMC11434005
- DOI: 10.3390/molecules29184361
Metal-Based Drug-DNA Interactions and Analytical Determination Methods
Abstract
DNA structure has many potential places where endogenous compounds and xenobiotics can bind. Therefore, xenobiotics bind along the sites of the nucleic acid with the aim of changing its structure, its genetic message, and, implicitly, its functions. Currently, there are several mechanisms known to be involved in DNA binding. These mechanisms are covalent and non-covalent interactions. The covalent interaction or metal base coordination is an irreversible binding and it is represented by an intra-/interstrand cross-link. The non-covalent interaction is generally a reversible binding and it is represented by intercalation between DNA base pairs, insertion, major and/or minor groove binding, and electrostatic interactions with the sugar phosphate DNA backbone. In the present review, we focus on the types of DNA-metal complex interactions (including some representative examples) and on presenting the methods currently used to study them.
Keywords: DNA; DNA interactions; bioinorganic chemistry; metal complexes.
Conflict of interest statement
The authors declare no conflicts of interest.
Figures
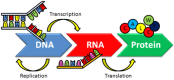
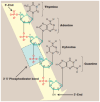
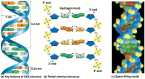
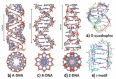
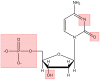
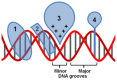
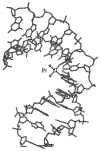

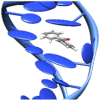
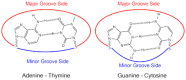
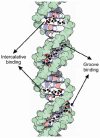
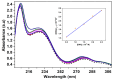
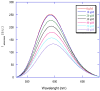
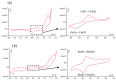
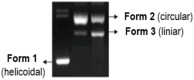
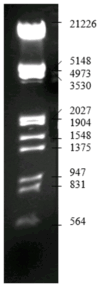
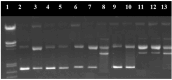
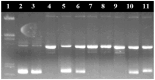
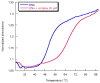
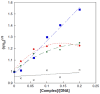
Similar articles
-
Metal complex interactions with DNA.Dalton Trans. 2015 Feb 28;44(8):3505-26. doi: 10.1039/c4dt02700k. Dalton Trans. 2015. PMID: 25427534
-
Raman spectroscopy of DNA-metal complexes. I. Interactions and conformational effects of the divalent cations: Mg, Ca, Sr, Ba, Mn, Co, Ni, Cu, Pd, and Cd.Biophys J. 1993 Nov;65(5):1916-28. doi: 10.1016/S0006-3495(93)81263-3. Biophys J. 1993. PMID: 8298021 Free PMC article.
-
Long range 1,4 and 1,6-interstrand cross-links formed by a trinuclear platinum complex. Minor groove preassociation affects kinetics and mechanism of cross-link formation as well as adduct structure.J Am Chem Soc. 2004 Feb 25;126(7):2166-80. doi: 10.1021/ja036105u. J Am Chem Soc. 2004. PMID: 14971952
-
Studying non-covalent drug-DNA interactions.Arch Biochem Biophys. 2015 Jun 15;576:49-60. doi: 10.1016/j.abb.2015.03.024. Epub 2015 May 5. Arch Biochem Biophys. 2015. PMID: 25951786 Review.
-
From cisplatin to artificial nucleases--the role of metal ion-nucleic acid interactions in biology.Biometals. 1992 Winter;5(4):195-208. doi: 10.1007/BF01061218. Biometals. 1992. PMID: 1463927 Review.
References
-
- Odani A. Inorganic biochemistry. In: Yamaguchi T., Persson I., editors. Metal Ions and Complexes in Solution. Volume 2 Royal Society of Chemistry; London, UK: 2023.
-
- Hadjiliadis N., Sletten E. Metal Complexes—DNA Interactions. Wiley; Hoboken, NJ, USA: 2009.
-
- Muthaiah S., Bhatia A., Kannan M. Stability of metal complexes. In: Srivastva A.N., editor. Stability and Applications of Coordination Copounds. IntechOpen; London, UK: 2020.
Publication types
MeSH terms
Substances
Grants and funding
LinkOut - more resources
Full Text Sources