Altered metabolism in cancer: insights into energy pathways and therapeutic targets
- PMID: 39294640
- PMCID: PMC11409553
- DOI: 10.1186/s12943-024-02119-3
Altered metabolism in cancer: insights into energy pathways and therapeutic targets
Abstract
Cancer cells undergo significant metabolic reprogramming to support their rapid growth and survival. This study examines important metabolic pathways like glycolysis, oxidative phosphorylation, glutaminolysis, and lipid metabolism, focusing on how they are regulated and their contributions to the development of tumors. The interplay between oncogenes, tumor suppressors, epigenetic modifications, and the tumor microenvironment in modulating these pathways is examined. Furthermore, we discuss the therapeutic potential of targeting cancer metabolism, presenting inhibitors of glycolysis, glutaminolysis, the TCA cycle, fatty acid oxidation, LDH, and glucose transport, alongside emerging strategies targeting oxidative phosphorylation and lipid synthesis. Despite the promise, challenges such as metabolic plasticity and the need for combination therapies and robust biomarkers persist, underscoring the necessity for continued research in this dynamic field.
Keywords: Cancer metabolism; Glutaminolysis; Glycolysis; Lipid metabolism; Oxidative phosphorylation.
© 2024. The Author(s).
Conflict of interest statement
The authors declare no competing interests.
Figures
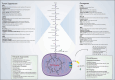
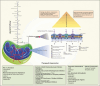
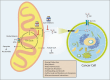
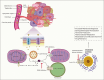
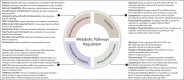
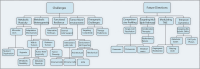
Similar articles
-
The dichotomous role of the glycolytic metabolism pathway in cancer metastasis: Interplay with the complex tumor microenvironment and novel therapeutic strategies.Semin Cancer Biol. 2020 Feb;60:238-248. doi: 10.1016/j.semcancer.2019.08.025. Epub 2019 Aug 21. Semin Cancer Biol. 2020. PMID: 31445217 Review.
-
Metabolic reprogramming in the tumour microenvironment: a hallmark shared by cancer cells and T lymphocytes.Immunology. 2017 Oct;152(2):175-184. doi: 10.1111/imm.12777. Epub 2017 Jul 10. Immunology. 2017. PMID: 28621843 Free PMC article. Review.
-
The emerging role of targeting cancer metabolism for cancer therapy.Tumour Biol. 2020 Oct;42(10):1010428320965284. doi: 10.1177/1010428320965284. Tumour Biol. 2020. PMID: 33028168 Review.
-
Metabolic Rewiring in Cancer: Small Molecule Inhibitors in Colorectal Cancer Therapy.Molecules. 2024 May 2;29(9):2110. doi: 10.3390/molecules29092110. Molecules. 2024. PMID: 38731601 Free PMC article. Review.
-
Drug discovery strategies in the field of tumor energy metabolism: Limitations by metabolic flexibility and metabolic resistance to chemotherapy.Biochim Biophys Acta Bioenerg. 2017 Aug;1858(8):674-685. doi: 10.1016/j.bbabio.2017.02.005. Epub 2017 Feb 16. Biochim Biophys Acta Bioenerg. 2017. PMID: 28213330 Review.
References
-
- Sedeta E, Sung H, Laversanne M, Bray F, Jemal A. Recent mortality patterns and time trends for the major cancers in 47 countries worldwide. Cancer Epidemiol Biomarkers Prev. 2023;32:894–905. - PubMed
-
- Kim Y, Jang Y, Kim MS, Kang C. Metabolic remodeling in cancer and senescence and its therapeutic implications. Trends Endocrinol Metab. 2024;35:744. - PubMed
Publication types
MeSH terms
Substances
Grants and funding
LinkOut - more resources
Full Text Sources