Predictive power of deleterious single amino acid changes to infer on AAV2 and AAV2-13 capsids fitness
- PMID: 39286333
- PMCID: PMC11403266
- DOI: 10.1016/j.omtm.2024.101327
Predictive power of deleterious single amino acid changes to infer on AAV2 and AAV2-13 capsids fitness
Abstract
Adeno-associated virus (AAV) is the most widely used vector for in vivo gene transfer. A major limitation of capsid engineering is the incomplete understanding of the consequences of multiple amino acid variations on AAV capsid stability resulting in high frequency of non-viable capsids. In this context, the study of natural AAV variants can provide valuable insights into capsid regions that exhibit greater tolerance to mutations. Here, the characterization of AAV2 variants and the analysis of two public capsid libraries highlighted common features associated with deleterious mutations, suggesting that the impact of mutations on capsid viability is strictly dependent on their 3D location within the capsid structure. We developed a novel prediction method to infer the fitness of AAV2 variants containing multiple amino acid variations with 98% sensitivity, 98% accuracy, and 95% specificity. This novel approach might streamline the development of AAV vector libraries enriched in viable capsids, thus accelerating the identification of therapeutic candidates among engineered capsids.
Keywords: AAV vector; AAV viability; adeno-associated virus; capsid engineering; deleterious mutation; fitness; hyper variable regions; prediction model; wild-type variant.
© 2024 The Author(s).
Conflict of interest statement
T.L.B., P.V., J.N., G.R., S.I., J.-C.N., and J.Z.-R. are authors in patents related to capsids development. G.R. is associate editor of the journal Molecular Therapy Methods & Clinical Development.
Figures
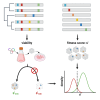
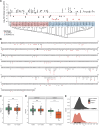
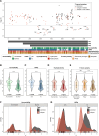
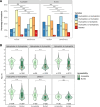
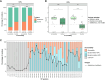
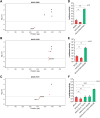
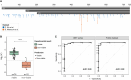
Similar articles
-
Structural and Biophysical Analysis of Adeno-Associated Virus Serotype 2 Capsid Assembly Variants.J Virol. 2023 Jul 27;97(7):e0177222. doi: 10.1128/jvi.01772-22. Epub 2023 Jun 13. J Virol. 2023. PMID: 37310260 Free PMC article.
-
Improved Genome Packaging Efficiency of Adeno-associated Virus Vectors Using Rep Hybrids.J Virol. 2021 Sep 9;95(19):e0077321. doi: 10.1128/JVI.00773-21. Epub 2021 Jul 21. J Virol. 2021. PMID: 34287038 Free PMC article.
-
Relevance of Assembly-Activating Protein for Adeno-associated Virus Vector Production and Capsid Protein Stability in Mammalian and Insect Cells.J Virol. 2017 Sep 27;91(20):e01198-17. doi: 10.1128/JVI.01198-17. Print 2017 Oct 15. J Virol. 2017. PMID: 28768875 Free PMC article.
-
The role of the adeno-associated virus capsid in gene transfer.Methods Mol Biol. 2008;437:51-91. doi: 10.1007/978-1-59745-210-6_2. Methods Mol Biol. 2008. PMID: 18369962 Free PMC article. Review.
-
Adeno-associated virus serotypes for gene therapeutics.Curr Opin Pharmacol. 2015 Oct;24:59-67. doi: 10.1016/j.coph.2015.07.006. Epub 2015 Aug 25. Curr Opin Pharmacol. 2015. PMID: 26291407 Review.
References
LinkOut - more resources
Full Text Sources