PTN from Leydig cells activates SDC2 and modulates human spermatogonial stem cell proliferation and survival via GFRA1
- PMID: 39285301
- PMCID: PMC11406790
- DOI: 10.1186/s40659-024-00546-6
PTN from Leydig cells activates SDC2 and modulates human spermatogonial stem cell proliferation and survival via GFRA1
Abstract
Background: Spermatogonial stem cells (SSCs) are essential for the maintenance and initiation of male spermatogenesis. Despite the advances in understanding SSC biology in mouse models, the mechanisms underlying human SSC development remain elusive.
Results: Here, we analyzed the signaling pathways involved in SSC regulation by testicular somatic cells using single-cell sequencing data (GEO datasets: GSE149512 and GSE112013) and identified that Leydig cells communicate with SSCs through pleiotrophin (PTN) and its receptor syndecan-2 (SDC2). Immunofluorescence, STRING prediction, and protein immunoprecipitation assays confirmed the interaction between PTN and SDC2 in spermatogonia, but their co-localization was observed only in approximately 50% of the cells. The knockdown of SDC2 in human SSC lines impaired cell proliferation, DNA synthesis, and the expression of PLZF, a key marker for SSC self-renewal. Transcriptome analysis revealed that SDC2 knockdown downregulated the expression of GFRA1, a crucial factor for SSC proliferation and self-renewal, and inhibited the HIF-1 signaling pathway. Exogenous PTN rescued the proliferation and GFRA1 expression in SDC2 knockdown SSC lines. In addition, we found downregulation of PTN and SDC2 as well as altered localization in non-obstructive azoospermia (NOA) patients, suggesting that downregulation of PTN and SDC2 may be associated with impaired spermatogenesis.
Conclusions: Our results uncover a novel mechanism of human SSC regulation by the testicular microenvironment and suggest a potential therapeutic target for male infertility.
Keywords: Apoptosis; Pleiotrophin; Proliferation; Self-renewal; Spermatogonial stem cells; Syndecan-2.
© 2024. The Author(s).
Conflict of interest statement
No authors declare competing interests.
Figures
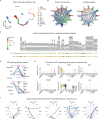
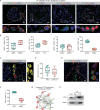
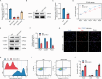
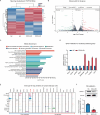
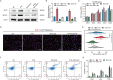
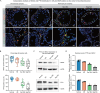
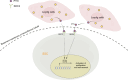
Similar articles
-
Glial cell-line derived neurotrophic factor-mediated RET signaling regulates spermatogonial stem cell fate.Biol Reprod. 2006 Feb;74(2):314-21. doi: 10.1095/biolreprod.105.047365. Epub 2005 Oct 19. Biol Reprod. 2006. PMID: 16237148
-
Gdnf-Gfra1 pathway is expressed in a spermatogenetic-dependent manner and is regulated by Fsh in a fish testis.Biol Reprod. 2014 Oct;91(4):94. doi: 10.1095/biolreprod.114.119834. Epub 2014 Aug 27. Biol Reprod. 2014. PMID: 25165121
-
Loss of Etv5 decreases proliferation and RET levels in neonatal mouse testicular germ cells and causes an abnormal first wave of spermatogenesis.Biol Reprod. 2009 Aug;81(2):258-66. doi: 10.1095/biolreprod.108.075200. Epub 2009 Apr 15. Biol Reprod. 2009. PMID: 19369650 Free PMC article.
-
Noncoding RNAs: Potential players in the self-renewal of mammalian spermatogonial stem cells.Mol Reprod Dev. 2018 Aug;85(8-9):720-728. doi: 10.1002/mrd.23041. Epub 2018 Aug 16. Mol Reprod Dev. 2018. PMID: 29969526 Review.
-
Cellular Therapy via Spermatogonial Stem Cells for Treating Impaired Spermatogenesis, Non-Obstructive Azoospermia.Cells. 2021 Jul 14;10(7):1779. doi: 10.3390/cells10071779. Cells. 2021. PMID: 34359947 Free PMC article. Review.
References
-
- Qiao J, Wang Y, Li X, Jiang F, Zhang Y, Ma J, et al. A Lancet Commission on 70 years of women’s reproductive, maternal, newborn, child, and adolescent health in China. Lancet. 2021;397(10293):2497–536. - PubMed
-
- Agarwal A, Baskaran S, Parekh N, Cho CL, Henkel R, Vij S, et al. Male infertility. Lancet. 2021;397(10271):319–33. - PubMed
-
- Saitou M, Hayashi K. Mammalian in vitro gametogenesis. Sci (New York NY). 2021;374(6563):eaaz6830. - PubMed
MeSH terms
Substances
Grants and funding
- 82201771/National Natural Science Foundation for Young Scholars of China
- 2024JJ6083/Natural Science Foundation of Hunan Province
- 2023JJ31018/Natural Science Foundation of Hunan Province
- W20243143/Health Research Project of Hunan Provincial Health Commission
- 20231769/Health Research Project of Hunan Provincial Health Commission
LinkOut - more resources
Full Text Sources