Spatial organization of translation and translational repression in two phases of germ granules
- PMID: 39271704
- PMCID: PMC11399267
- DOI: 10.1038/s41467-024-52346-x
Spatial organization of translation and translational repression in two phases of germ granules
Abstract
Most RNA-protein condensates are composed of heterogeneous immiscible phases. However, how this multiphase organization contributes to their biological functions remains largely unexplored. Drosophila germ granules, a class of RNA-protein condensates, are the site of mRNA storage and translational activation. Here, using super-resolution microscopy and single-molecule imaging approaches, we show that germ granules have a biphasic organization and that translation occurs in the outer phase and at the surface of the granules. The localization, directionality, and compaction of mRNAs within the granule depend on their translation status, translated mRNAs being enriched in the outer phase with their 5'end oriented towards the surface. Translation is strongly reduced when germ granule biphasic organization is lost. These findings reveal the intimate links between the architecture of RNA-protein condensates and the organization of their different functions, highlighting the functional compartmentalization of these condensates.
© 2024. The Author(s).
Conflict of interest statement
The authors declare no competing interests.
Figures
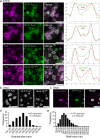
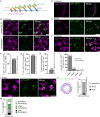
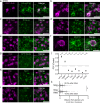
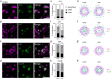
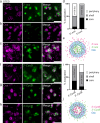
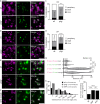
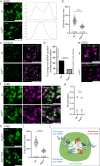
Similar articles
-
Direct observation of translational activation by a ribonucleoprotein granule.Nat Cell Biol. 2024 Aug;26(8):1322-1335. doi: 10.1038/s41556-024-01452-5. Epub 2024 Jul 4. Nat Cell Biol. 2024. PMID: 38965420 Free PMC article.
-
Proteins rather than mRNAs regulate nucleation and persistence of Oskar germ granules in Drosophila.Cell Rep. 2023 Jul 25;42(7):112723. doi: 10.1016/j.celrep.2023.112723. Epub 2023 Jun 28. Cell Rep. 2023. PMID: 37384531 Free PMC article.
-
Germ granules in Drosophila.Traffic. 2019 Sep;20(9):650-660. doi: 10.1111/tra.12674. Epub 2019 Jul 31. Traffic. 2019. PMID: 31218815 Free PMC article. Review.
-
Stochastic Seeding Coupled with mRNA Self-Recruitment Generates Heterogeneous Drosophila Germ Granules.Curr Biol. 2018 Jun 18;28(12):1872-1881.e3. doi: 10.1016/j.cub.2018.04.037. Epub 2018 May 31. Curr Biol. 2018. PMID: 29861136 Free PMC article.
-
Germ granules and the control of mRNA translation.IUBMB Life. 2012 Jul;64(7):586-94. doi: 10.1002/iub.1039. Epub 2012 May 28. IUBMB Life. 2012. PMID: 22639345 Review.
Cited by
-
The mRNA dynamics underpinning translational control mechanisms of Drosophila melanogaster oogenesis.Biochem Soc Trans. 2024 Oct 30;52(5):2087-2099. doi: 10.1042/BST20231293. Biochem Soc Trans. 2024. PMID: 39263986 Free PMC article. Review.
References
MeSH terms
Substances
Grants and funding
LinkOut - more resources
Full Text Sources
Molecular Biology Databases