This is a preprint.
Centromeric transposable elements and epigenetic status drive karyotypic variation in the eastern hoolock gibbon
- PMID: 39257810
- PMCID: PMC11384015
- DOI: 10.1101/2024.08.29.610280
Centromeric transposable elements and epigenetic status drive karyotypic variation in the eastern hoolock gibbon
Abstract
Great apes have maintained a stable karyotype with few large-scale rearrangements; in contrast, gibbons have undergone a high rate of chromosomal rearrangements coincident with rapid centromere turnover. Here we characterize assembled centromeres in the Eastern hoolock gibbon, Hoolock leuconedys (HLE), finding a diverse group of transposable elements (TEs) that differ from the canonical alpha satellites found across centromeres of other apes. We find that HLE centromeres contain a CpG methylation centromere dip region, providing evidence this epigenetic feature is conserved in the absence of satellite arrays; nevertheless, we report a variety of atypical centromeric features, including protein-coding genes and mismatched replication timing. Further, large structural variations define HLE centromeres and distinguish them from other gibbons. Combined with differentially methylated TEs, topologically associated domain boundaries, and segmental duplications at chromosomal breakpoints, we propose that a "perfect storm" of multiple genomic attributes with propensities for chromosome instability shaped gibbon centromere evolution.
Keywords: centromeres; chromosome evolution; genome assembly; gibbons; methylation; primate genomics; replication timing; transposable elements.
Conflict of interest statement
Declaration of Interests R.J.O. serves on the SAB of Colossal Biosciences and has been supported to present at ONT events.
Figures
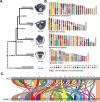
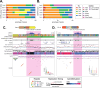
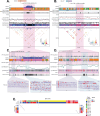
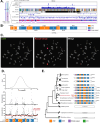
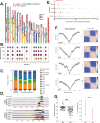
Similar articles
-
Comparative Analyses of Gibbon Centromeres Reveal Dynamic Genus-Specific Shifts in Repeat Composition.Mol Biol Evol. 2021 Aug 23;38(9):3972-3992. doi: 10.1093/molbev/msab148. Mol Biol Evol. 2021. PMID: 33983366 Free PMC article.
-
Centromere remodeling in Hoolock leuconedys (Hylobatidae) by a new transposable element unique to the gibbons.Genome Biol Evol. 2012;4(7):648-58. doi: 10.1093/gbe/evs048. Epub 2012 May 16. Genome Biol Evol. 2012. PMID: 22593550 Free PMC article.
-
Tandem repeat sequences evolutionarily related to SVA-type retrotransposons are expanded in the centromere region of the western hoolock gibbon, a small ape.J Hum Genet. 2012 Dec;57(12):760-5. doi: 10.1038/jhg.2012.107. Epub 2012 Aug 30. J Hum Genet. 2012. PMID: 22931862
-
Centromere Repeats: Hidden Gems of the Genome.Genes (Basel). 2019 Mar 16;10(3):223. doi: 10.3390/genes10030223. Genes (Basel). 2019. PMID: 30884847 Free PMC article. Review.
-
Transposable elements: genome innovation, chromosome diversity, and centromere conflict.Chromosome Res. 2018 Mar;26(1-2):5-23. doi: 10.1007/s10577-017-9569-5. Epub 2018 Jan 13. Chromosome Res. 2018. PMID: 29332159 Free PMC article. Review.
References
-
- Misceo D., Capozzi O., Roberto R., Dell’oglio M.P., Rocchi M., Stanyon R., and Archidiacono N. (2008). Tracking the complex flow of chromosome rearrangements from the Hominoidea Ancestor to extant Hylobates and Nomascus Gibbons by high-resolution synteny mapping. Genome Res. 18, 1530–1537. - PMC - PubMed
-
- Mittermeier R.A., Wilson D.E., and Rylands A.B. (2013). Handbook of the Mammals of the World: Primates (Lynx Edicions; ).
Publication types
Grants and funding
LinkOut - more resources
Full Text Sources