Zinc dampens antitumor immunity by promoting Foxp3+ regulatory T cells
- PMID: 39247196
- PMCID: PMC11377231
- DOI: 10.3389/fimmu.2024.1389387
Zinc dampens antitumor immunity by promoting Foxp3+ regulatory T cells
Abstract
Introduction: The role of zinc (Zn) in tumor development and immune modulation has always been paradoxical. This study redefines our understanding of the impact of Zn on cancer progression and therapeutic strategies.
Methods: We investigated the effects of dietary Zn levels on tumor progression and immune responses. This included examining the impact of both high and deficient dietary Zn, as well as Zn chelation, on tumor growth and immune cell populations. Specifically, we analyzed the frequency of Foxp3+ regulatory T-cells (Tregs) and identified the role of FOXO1 in Zn-mediated effects on Tregs. Additionally, we explored the therapeutic potential of clioquinol (CQ) in enhancing α-PD-1 immunotherapy responses, particularly in melanoma.
Results: Our findings show that high dietary Zn promotes tumor progression by fostering a protumorigenic environment mediated by T cells. Increased Zn intake was found to facilitate tumor progression by increasing Foxp3+ Treg frequency. In contrast, deficiency in dietary Zn and chelation of tissue Zn emerged as potent drivers of antitumor immunity. We pinpointed FOXO1 as the master regulator governing the influence of Zn on Tregs.
Discussion: These results reveal a novel mechanistic insight into how Zn influences tumor progression and immune regulation. The identification of FOXO1 as a key regulator opens new avenues for understanding the role of Zn in cancer biology. Furthermore, we introduce a promising therapeutic approach by showing that administering clioquinol (CQ) significantly enhances α-PD-1 immunotherapy response, particularly in melanoma. These revelations transform our comprehension of the multifaceted role of Zn in tumorigenesis and immune regulation, highlighting innovative possibilities for cancer therapy.
Keywords: FOXO1; PD-1; antitumor immunity; cancer; checkpoint inhibition therapy; regulatory T cell; zinc.
Copyright © 2024 Narayan, Dalal, Rizvi and Awasthi.
Conflict of interest statement
The authors declare that the research was conducted in the absence of any commercial or financial relationships that could be construed as a potential conflict of interest.
Figures
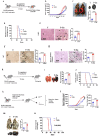
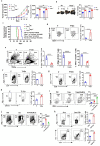
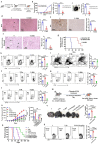
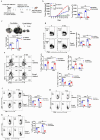
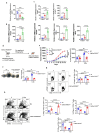
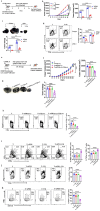
Similar articles
-
INFα-2b inhibitory effects on CD4(+)CD25(+)FOXP3(+) regulatory T cells in the tumor microenvironment of C57BL/6 J mice with melanoma xenografts.BMC Cancer. 2016 Jul 7;16:397. doi: 10.1186/s12885-016-2473-0. BMC Cancer. 2016. PMID: 27389040 Free PMC article.
-
Remodeling of the tumor microenvironment via disrupting Blimp1+ effector Treg activity augments response to anti-PD-1 blockade.Mol Cancer. 2021 Nov 20;20(1):150. doi: 10.1186/s12943-021-01450-3. Mol Cancer. 2021. PMID: 34798898 Free PMC article.
-
Prophylactic DNA vaccine targeting Foxp3+ regulatory T cells depletes myeloid-derived suppressor cells and improves anti-melanoma immune responses in a murine model.Cancer Immunol Immunother. 2018 Mar;67(3):367-379. doi: 10.1007/s00262-017-2088-6. Epub 2017 Nov 9. Cancer Immunol Immunother. 2018. PMID: 29124314 Free PMC article.
-
FoxP3+ T regulatory cells in cancer: Prognostic biomarkers and therapeutic targets.Cancer Lett. 2020 Oct 10;490:174-185. doi: 10.1016/j.canlet.2020.07.022. Epub 2020 Jul 25. Cancer Lett. 2020. PMID: 32721551 Review.
-
Roles of regulatory T cells in cancer immunity.Int Immunol. 2016 Aug;28(8):401-9. doi: 10.1093/intimm/dxw025. Epub 2016 May 9. Int Immunol. 2016. PMID: 27160722 Free PMC article. Review.
References
MeSH terms
Substances
Grants and funding
LinkOut - more resources
Full Text Sources
Research Materials
Miscellaneous