This is a preprint.
Drosophila Clueless ribonucleoprotein particles display novel dynamics that rely on the availability of functional protein and polysome equilibrium
- PMID: 39229069
- PMCID: PMC11370489
- DOI: 10.1101/2024.08.21.609023
Drosophila Clueless ribonucleoprotein particles display novel dynamics that rely on the availability of functional protein and polysome equilibrium
Abstract
The cytoplasm is populated with many ribonucleoprotein (RNP) particles that post-transcriptionally regulate mRNAs. These membraneless organelles assemble and disassemble in response to stress, performing functions such as sequestering stalled translation pre-initiation complexes or mRNA storage, repression and decay. Drosophila Clueless (Clu) is a conserved multi-domain ribonucleoprotein essential for mitochondrial function that forms dynamic particles within the cytoplasm. Unlike well-known RNP particles, stress granules and Processing bodies, Clu particles completely disassemble under nutritional or oxidative stress. However, it is poorly understood how disrupting protein synthesis affects Clu particle dynamics, especially since Clu binds mRNA and ribosomes. Here, we capitalize on ex vivo and in vivo imaging of Drosophila female germ cells to determine what domains of Clu are necessary for Clu particle assembly, how manipulating translation using translation inhibitors affects particle dynamics, and how Clu particle movement relates to mitochondrial association. Using Clu deletion analysis and live and fixed imaging, we identified three protein domains in Clu, which are essential for particle assembly. In addition, we demonstrated that overexpressing functional Clu disassembled particles, while overexpression of deletion constructs did not. To examine how decreasing translation affects particle dynamics, we inhibited translation in Drosophila germ cells using cycloheximide and puromycin. In contrast to stress granules and Processing bodies, cycloheximide treatment did not disassemble Clu particles yet puromycin treatment did. Surprisingly, cycloheximide stabilized particles in the presence of oxidative and nutritional stress. These findings demonstrate that Clu particles have novel dynamics in response to altered ribosome activity compared to stress granules and Processing bodies and support a model where they function as hubs of translation whose assembly heavily depends on the dynamic availability of polysomes.
Conflict of interest statement
Conflict of interest: The authors declare that they have no conflicts of interest with the contents of this article.
Figures
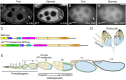
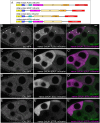
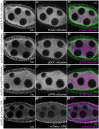
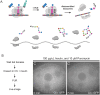
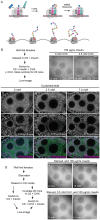
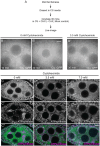
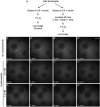
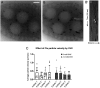
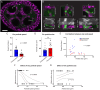
Similar articles
-
Using Experience Sampling Methodology to Capture Disclosure Opportunities for Autistic Adults.Autism Adulthood. 2023 Dec 1;5(4):389-400. doi: 10.1089/aut.2022.0090. Epub 2023 Dec 12. Autism Adulthood. 2023. PMID: 38116059 Free PMC article.
-
Depressing time: Waiting, melancholia, and the psychoanalytic practice of care.In: Kirtsoglou E, Simpson B, editors. The Time of Anthropology: Studies of Contemporary Chronopolitics. Abingdon: Routledge; 2020. Chapter 5. In: Kirtsoglou E, Simpson B, editors. The Time of Anthropology: Studies of Contemporary Chronopolitics. Abingdon: Routledge; 2020. Chapter 5. PMID: 36137063 Free Books & Documents. Review.
-
"I've Spent My Whole Life Striving to Be Normal": Internalized Stigma and Perceived Impact of Diagnosis in Autistic Adults.Autism Adulthood. 2023 Dec 1;5(4):423-436. doi: 10.1089/aut.2022.0066. Epub 2023 Dec 12. Autism Adulthood. 2023. PMID: 38116050 Free PMC article.
-
"It Is a Big Spider Web of Things": Sensory Experiences of Autistic Adults in Public Spaces.Autism Adulthood. 2023 Dec 1;5(4):411-422. doi: 10.1089/aut.2022.0024. Epub 2023 Dec 12. Autism Adulthood. 2023. PMID: 38116051 Free PMC article.
-
Plasmapheresis to remove amyloid fibrin(ogen) particles for treating the post-COVID-19 condition.Cochrane Database Syst Rev. 2023 Jul 26;7(7):CD015775. doi: 10.1002/14651858.CD015775. Cochrane Database Syst Rev. 2023. PMID: 37491597 Free PMC article. Review.
References
Publication types
Grants and funding
LinkOut - more resources
Full Text Sources
Research Materials
Miscellaneous