NeuroAiDTM-II (MLC901) Promoted Neurogenesis by Activating the PI3K/AKT/GSK-3β Signaling Pathway in Rat Spinal Cord Injury Models
- PMID: 39200383
- PMCID: PMC11352105
- DOI: 10.3390/biomedicines12081920
NeuroAiDTM-II (MLC901) Promoted Neurogenesis by Activating the PI3K/AKT/GSK-3β Signaling Pathway in Rat Spinal Cord Injury Models
Abstract
Traumatic damage to the spinal cord (SCI) frequently leads to irreversible neurological deficits, which may be related to apoptotic neurodegeneration in nerve tissue. The MLC901 treatment possesses neuroprotective and neuroregenerative activity. This study aimed to explore the regenerative potential of MLC901 and the molecular mechanisms promoting neurogenesis and functional recovery after SCI in rats. A calibrated forceps compression injury for 15 s was used to induce SCI in rats, followed by an examination of the impacts of MLC901 on functional recovery. The Basso, Beattie, and Bresnahan (BBB) scores were utilized to assess neuronal functional recovery; H&E and immunohistochemistry (IHC) staining were also used to observe pathological changes in the lesion area. Somatosensory Evoked Potentials (SEPs) were measured using the Nicolet® Viking Quest™ apparatus. Additionally, we employed the Western blot assay to identify PI3K/AKT/GSK-3β pathway-related proteins and to assess the levels of GAP-43 and GFAP through immunohistochemistry staining. The study findings revealed that MLC901 improved hind-limb motor function recovery, alleviating the pathological damage induced by SCI. Moreover, MLC901 significantly enhanced locomotor activity, SEPs waveform, latency, amplitude, and nerve conduction velocity. The treatment also promoted GAP-43 expression and reduced reactive astrocytes (GFAP). MLC901 treatment activated p-AKT reduced p-GSK-3β expression levels and showed a normalized ratio (fold changes) relative to β-tubulin. Specifically, p-AKT exhibited a 4-fold increase, while p-GSK-3β showed a 2-fold decrease in T rats compared to UT rats. In conclusion, these results suggest that the treatment mitigates pathological tissue damage and effectively improves neural functional recovery following SCI, primarily by alleviating apoptosis and promoting neurogenesis. The underlying molecular mechanism of this treatment mainly involves the activation of the PI3K/AKT/GSK-3β pathway.
Keywords: GAP-43; GFAP; MLC901 (NeuroAiDTM-II); calibrated forceps compression injury; rat mechanical spinal injury model; signaling pathway.
Conflict of interest statement
The authors declare no conflicts of interest in preparing this article.
Figures
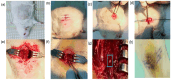
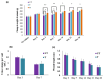
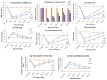
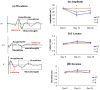
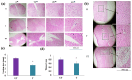
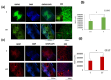
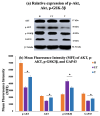
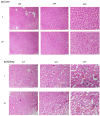
Similar articles
-
Astaxanthin alleviates spinal cord ischemia-reperfusion injury via activation of PI3K/Akt/GSK-3β pathway in rats.J Orthop Surg Res. 2020 Jul 23;15(1):275. doi: 10.1186/s13018-020-01790-8. J Orthop Surg Res. 2020. PMID: 32703256 Free PMC article.
-
Local Delivery of β-Elemene Improves Locomotor Functional Recovery by Alleviating Endoplasmic Reticulum Stress and Reducing Neuronal Apoptosis in Rats with Spinal Cord Injury.Cell Physiol Biochem. 2018;49(2):595-609. doi: 10.1159/000492996. Epub 2018 Aug 30. Cell Physiol Biochem. 2018. PMID: 30165357
-
Quercetin reduces neural tissue damage and promotes astrocyte activation after spinal cord injury in rats.J Cell Biochem. 2018 Feb;119(2):2298-2306. doi: 10.1002/jcb.26392. Epub 2017 Oct 18. J Cell Biochem. 2018. PMID: 28865131
-
Combined application of Rho-ROCKII and GSK-3β inhibitors exerts an improved protective effect on axonal regeneration in rats with spinal cord injury.Mol Med Rep. 2016 Dec;14(6):5180-5188. doi: 10.3892/mmr.2016.5918. Epub 2016 Nov 1. Mol Med Rep. 2016. PMID: 27840930 Free PMC article.
-
Function of GSK‑3 signaling in spinal cord injury (Review).Exp Ther Med. 2023 Oct 3;26(5):541. doi: 10.3892/etm.2023.12240. eCollection 2023 Nov. Exp Ther Med. 2023. PMID: 37869638 Free PMC article. Review.
References
-
- Anjum A., Yazid M.D.i., Fauzi Daud M., Idris J., Ng A.M.H., Selvi Naicker A., Ismail O.H.R., Athi Kumar R.K., Lokanathan Y. Spinal cord injury: Pathophysiology, multimolecular interactions, and underlying recovery mechanisms. Int. J. Mol. Sci. 2020;21:7533. doi: 10.3390/ijms21207533. - DOI - PMC - PubMed
-
- Anjum A., Cheah Y.J., Yazid M.D.I., Daud M.F., Idris J., Ng M.H., Naicker A.S., Ismail O.H., Athi Kumar R.K., Tan G.C. Protocol paper: Kainic acid excitotoxicity-induced spinal cord injury paraplegia in Sprague–Dawley rats. Biol. Res. 2022;55:38. doi: 10.1186/s40659-022-00407-0. - DOI - PMC - PubMed
Grants and funding
LinkOut - more resources
Full Text Sources
Miscellaneous