Protection of Tight Junctional Complexes between hCMEC/D3 Cells by Deep-Sea Fibrinolytic Compound FGFC1
- PMID: 39195457
- PMCID: PMC11355241
- DOI: 10.3390/md22080341
Protection of Tight Junctional Complexes between hCMEC/D3 Cells by Deep-Sea Fibrinolytic Compound FGFC1
Abstract
Tight junctional complexes (TJCs) between cerebral microvascular endothelial cells (CMECs) are essential parts of the blood-brain barrier (BBB), whose regulation closely correlates to the BBB's integrity and function. hCMEC/D3 is the typical cell line used to imitate and investigate the barrier function of the BBB via the construction of an in vitro model. This study aims to investigate the protective effect of the deep-sea-derived fibrinolytic compound FGFC1 against H2O2-induced dysfunction of TJCs and to elucidate the underlying mechanism. The barrier function was shown to decline following exposure to 1 mM H2O2 in an in vitro model of hCMEC/D3 cells, with a decreasing temperature-corrected transendothelial electrical resistance (tcTEER) value. The decrease in the tcTEER value was significantly inhibited by 80 or 100 µM FGFC1, which suggested it efficiently protected the barrier integrity, allowing it to maintain its function against the H2O2-induced dysfunction. According to immunofluorescence microscopy (IFM) and quantitative real-time polymerase chain reaction (qRT-PCR), compared to the H2O2-treated group, 80~100 µM FGFC1 enhanced the expression of claudin-5 (CLDN-5) and VE-cadherin (VE-cad). And this enhancement was indicated to be mainly achieved by both up-regulation of CLDN-5 and inhibition of the down-regulation by H2O2 of VE-cad at the transcriptional level. Supported by FGFC1's molecular docking to these proteins with reasonable binding energy, FGFC1 was proved to exert a positive effect on TJCs' barrier function in hCMEC/D3 cells via targeting CLDN-5 and VE-cad. This is the first report on the protection against H2O2-induced barrier dysfunction by FGFC1 in addition to its thrombolytic effect. With CLDN-5 and VE-cad as the potential target proteins of FGFC1, this study provides evidence at the cellular and molecular levels for FGFC1's reducing the risk of bleeding transformation following its application in thrombolytic therapy for cerebral thrombosis.
Keywords: FGFC1; blood–brain barrier; deep-sea fibrinolytic compounds; thrombolytic therapy; tight junctional complexes.
Conflict of interest statement
The authors declare no conflicts of interest.
Figures
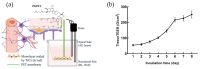
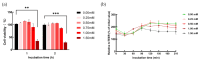
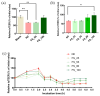
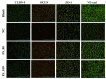
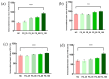
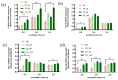
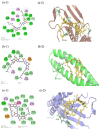
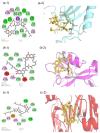
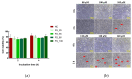
Similar articles
-
Zika Virus NS1 Suppresses VE-Cadherin and Claudin-5 via hsa-miR-101-3p in Human Brain Microvascular Endothelial Cells.Mol Neurobiol. 2021 Dec;58(12):6290-6303. doi: 10.1007/s12035-021-02548-x. Epub 2021 Sep 6. Mol Neurobiol. 2021. PMID: 34487317
-
Differential effects of hydrocortisone and TNFalpha on tight junction proteins in an in vitro model of the human blood-brain barrier.J Physiol. 2008 Apr 1;586(7):1937-49. doi: 10.1113/jphysiol.2007.146852. Epub 2008 Feb 7. J Physiol. 2008. PMID: 18258663 Free PMC article.
-
Neurons enhance blood-brain barrier function via upregulating claudin-5 and VE-cadherin expression due to glial cell line-derived neurotrophic factor secretion.Elife. 2024 Oct 30;13:RP96161. doi: 10.7554/eLife.96161. Elife. 2024. PMID: 39475379 Free PMC article.
-
Altered Nrf2 signaling mediates hypoglycemia-induced blood-brain barrier endothelial dysfunction in vitro.PLoS One. 2015 Mar 25;10(3):e0122358. doi: 10.1371/journal.pone.0122358. eCollection 2015. PLoS One. 2015. PMID: 25807533 Free PMC article.
-
The CLDN5 gene at the blood-brain barrier in health and disease.Fluids Barriers CNS. 2023 Mar 28;20(1):22. doi: 10.1186/s12987-023-00424-5. Fluids Barriers CNS. 2023. PMID: 36978081 Free PMC article. Review.
Cited by
-
Novel Deep Sea Isoindole Alkaloid FGFC1 Exhibits Its Fibrinolytic Effects by Inhibiting Thrombin-Activatable Fibrinolysis Inhibitor.Pharmaceuticals (Basel). 2024 Oct 20;17(10):1401. doi: 10.3390/ph17101401. Pharmaceuticals (Basel). 2024. PMID: 39459040 Free PMC article.
References
MeSH terms
Substances
Grants and funding
LinkOut - more resources
Full Text Sources
Miscellaneous