Recent Advances in Nanomaterials for Modulation of Stem Cell Differentiation and Its Therapeutic Applications
- PMID: 39194636
- PMCID: PMC11352443
- DOI: 10.3390/bios14080407
Recent Advances in Nanomaterials for Modulation of Stem Cell Differentiation and Its Therapeutic Applications
Abstract
Challenges in directed differentiation and survival limit the clinical use of stem cells despite their promising therapeutic potential in regenerative medicine. Nanotechnology has emerged as a powerful tool to address these challenges and enable precise control over stem cell fate. In particular, nanomaterials can mimic an extracellular matrix and provide specific cues to guide stem cell differentiation and proliferation in the field of nanotechnology. For instance, recent studies have demonstrated that nanostructured surfaces and scaffolds can enhance stem cell lineage commitment modulated by intracellular regulation and external stimulation, such as reactive oxygen species (ROS) scavenging, autophagy, or electrical stimulation. Furthermore, nanoframework-based and upconversion nanoparticles can be used to deliver bioactive molecules, growth factors, and genetic materials to facilitate stem cell differentiation and tissue regeneration. The increasing use of nanostructures in stem cell research has led to the development of new therapeutic approaches. Therefore, this review provides an overview of recent advances in nanomaterials for modulating stem cell differentiation, including metal-, carbon-, and peptide-based strategies. In addition, we highlight the potential of these nano-enabled technologies for clinical applications of stem cell therapy by focusing on improving the differentiation efficiency and therapeutics. We believe that this review will inspire researchers to intensify their efforts and deepen their understanding, thereby accelerating the development of stem cell differentiation modulation, therapeutic applications in the pharmaceutical industry, and stem cell therapeutics.
Keywords: cellular adhesion; external stimulation; intracellular regulation; nanomaterials; stem cell differentiation.
Conflict of interest statement
The authors declare no conflicts of interest.
Figures
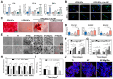
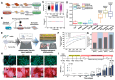
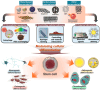
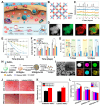
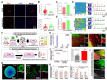
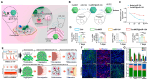
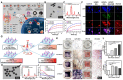
Similar articles
-
The potential of nanoparticles in stem cell differentiation and further therapeutic applications.Biotechnol J. 2016 Dec;11(12):1550-1560. doi: 10.1002/biot.201600453. Epub 2016 Oct 31. Biotechnol J. 2016. PMID: 27797150 Review.
-
Nanostructured scaffold as a determinant of stem cell fate.Stem Cell Res Ther. 2016 Dec 30;7(1):188. doi: 10.1186/s13287-016-0440-y. Stem Cell Res Ther. 2016. PMID: 28038681 Free PMC article. Review.
-
Nano-regenerative medicine towards clinical outcome of stem cell and tissue engineering in humans.J Cell Mol Med. 2012 Sep;16(9):1991-2000. doi: 10.1111/j.1582-4934.2012.01534.x. J Cell Mol Med. 2012. PMID: 22260258 Free PMC article. Review.
-
Nanomaterials for Engineering Stem Cell Responses.Adv Healthc Mater. 2015 Aug 5;4(11):1600-27. doi: 10.1002/adhm.201500272. Epub 2015 May 26. Adv Healthc Mater. 2015. PMID: 26010739 Review.
-
Nanotechnology-Based Approaches for Guiding Neural Regeneration.Acc Chem Res. 2016 Jan 19;49(1):17-26. doi: 10.1021/acs.accounts.5b00345. Epub 2015 Dec 14. Acc Chem Res. 2016. PMID: 26653885 Free PMC article.
References
Publication types
MeSH terms
Grants and funding
LinkOut - more resources
Full Text Sources
Medical