Simulation-driven design of stabilized SARS-CoV-2 spike S2 immunogens
- PMID: 39191724
- PMCID: PMC11350062
- DOI: 10.1038/s41467-024-50976-9
Simulation-driven design of stabilized SARS-CoV-2 spike S2 immunogens
Abstract
The full-length prefusion-stabilized SARS-CoV-2 spike (S) is the principal antigen of COVID-19 vaccines. Vaccine efficacy has been impacted by emerging variants of concern that accumulate most of the sequence modifications in the immunodominant S1 subunit. S2, in contrast, is the most evolutionarily conserved region of the spike and can elicit broadly neutralizing and protective antibodies. Yet, S2's usage as an alternative vaccine strategy is hampered by its general instability. Here, we use a simulation-driven approach to design S2-only immunogens stabilized in a closed prefusion conformation. Molecular simulations provide a mechanistic characterization of the S2 trimer's opening, informing the design of tryptophan substitutions that impart kinetic and thermodynamic stabilization. Structural characterization via cryo-EM shows the molecular basis of S2 stabilization in the closed prefusion conformation. Informed by molecular simulations and corroborated by experiments, we report an engineered S2 immunogen that exhibits increased protein expression, superior thermostability, and preserved immunogenicity against sarbecoviruses.
© 2024. The Author(s).
Conflict of interest statement
J.S.M., L.Z., R.E.A., X.N., L.C., and M.S. are inventors on a U.S. patent application describing the use of stabilized SARS-CoV-2 S proteins as vaccine antigens (Stabilized SARS-CoV-2 S Antigens, 63/583,090). K.C. is a member of the scientific advisory board of Integrum Scientific LLC and has consulted for Axon Advisors, LLC. K.C. owns shares in Integrum Scientific and Eitr Biologics, Inc. The other authors declare no competing interests.
Figures
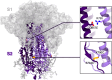
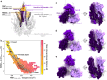
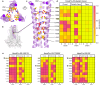
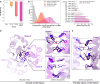
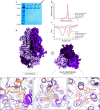
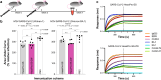
Similar articles
-
Safety and immunogenicity of a modified mRNA-lipid nanoparticle vaccine candidate against COVID-19: Results from a phase 1, dose-escalation study.Hum Vaccin Immunother. 2024 Dec 31;20(1):2408863. doi: 10.1080/21645515.2024.2408863. Epub 2024 Oct 18. Hum Vaccin Immunother. 2024. PMID: 39422261 Free PMC article. Clinical Trial.
-
SARS-CoV-2 BA.4/5 infection triggers more cross-reactive FcγRIIIa signaling and neutralization than BA.1, in the context of hybrid immunity.J Virol. 2024 Jul 23;98(7):e0067824. doi: 10.1128/jvi.00678-24. Epub 2024 Jul 2. J Virol. 2024. PMID: 38953380
-
Altered TMPRSS2 usage by SARS-CoV-2 Omicron impacts infectivity and fusogenicity.Nature. 2022 Mar;603(7902):706-714. doi: 10.1038/s41586-022-04474-x. Epub 2022 Feb 1. Nature. 2022. PMID: 35104837 Free PMC article.
-
The Key to Increase Immunogenicity of Next-Generation COVID-19 Vaccines Lies in the Inclusion of the SARS-CoV-2 Nucleocapsid Protein.J Immunol Res. 2024 May 29;2024:9313267. doi: 10.1155/2024/9313267. eCollection 2024. J Immunol Res. 2024. PMID: 38939745 Free PMC article. Review.
-
Depressing time: Waiting, melancholia, and the psychoanalytic practice of care.In: Kirtsoglou E, Simpson B, editors. The Time of Anthropology: Studies of Contemporary Chronopolitics. Abingdon: Routledge; 2020. Chapter 5. In: Kirtsoglou E, Simpson B, editors. The Time of Anthropology: Studies of Contemporary Chronopolitics. Abingdon: Routledge; 2020. Chapter 5. PMID: 36137063 Free Books & Documents. Review.
Cited by
-
Imprinting of serum neutralizing antibodies by Wuhan-1 mRNA vaccines.Nature. 2024 Jun;630(8018):950-960. doi: 10.1038/s41586-024-07539-1. Epub 2024 May 15. Nature. 2024. PMID: 38749479 Free PMC article.
-
Structural prediction of chimeric immunogens to elicit targeted antibodies against betacoronaviruses.bioRxiv [Preprint]. 2024 Sep 28:2023.01.31.526494. doi: 10.1101/2023.01.31.526494. bioRxiv. 2024. PMID: 36778336 Free PMC article. Preprint.
References
-
- World Health Organization. WHO COVID-19 Dashboard. https://covid19.who.int/ (2023).
MeSH terms
Substances
Grants and funding
LinkOut - more resources
Full Text Sources
Medical
Miscellaneous