A broadly cross-reactive i-body to AMA1 potently inhibits blood and liver stages of Plasmodium parasites
- PMID: 39174515
- PMCID: PMC11341838
- DOI: 10.1038/s41467-024-50770-7
A broadly cross-reactive i-body to AMA1 potently inhibits blood and liver stages of Plasmodium parasites
Abstract
Apical membrane antigen-1 (AMA1) is a conserved malarial vaccine candidate essential for the formation of tight junctions with the rhoptry neck protein (RON) complex, enabling Plasmodium parasites to invade human erythrocytes, hepatocytes, and mosquito salivary glands. Despite its critical role, extensive surface polymorphisms in AMA1 have led to strain-specific protection, limiting the success of AMA1-based interventions beyond initial clinical trials. Here, we identify an i-body, a humanised single-domain antibody-like molecule that recognises a conserved pan-species conformational epitope in AMA1 with low nanomolar affinity and inhibits the binding of the RON2 ligand to AMA1. Structural characterisation indicates that the WD34 i-body epitope spans the centre of the conserved hydrophobic cleft in AMA1, where interacting residues are highly conserved among all Plasmodium species. Furthermore, we show that WD34 inhibits merozoite invasion of erythrocytes by multiple Plasmodium species and hepatocyte invasion by P. falciparum sporozoites. Despite a short half-life in mouse serum, we demonstrate that WD34 transiently suppressed P. berghei infections in female BALB/c mice. Our work describes the first pan-species AMA1 biologic with inhibitory activity against multiple life-cycle stages of Plasmodium. With improved pharmacokinetic characteristics, WD34 could be a potential immunotherapy against multiple species of Plasmodium.
© 2024. The Author(s).
Conflict of interest statement
This study was a collaboration between AdAlta Limited and La Trobe University. M.F. is the founding chief scientist and a shareholder in AdAlta Ltd., and R.F.A. is also a shareholder in AdAlta. The other authors have declared no competing interests.
Figures
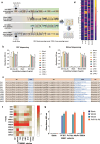
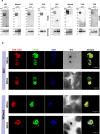
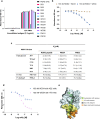
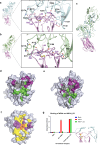
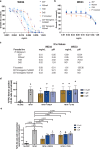
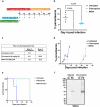
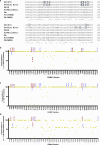
Similar articles
-
Overcoming antigenic diversity by enhancing the immunogenicity of conserved epitopes on the malaria vaccine candidate apical membrane antigen-1.PLoS Pathog. 2013;9(12):e1003840. doi: 10.1371/journal.ppat.1003840. Epub 2013 Dec 26. PLoS Pathog. 2013. PMID: 24385910 Free PMC article.
-
Immunization with a functional protein complex required for erythrocyte invasion protects against lethal malaria.Proc Natl Acad Sci U S A. 2014 Jul 15;111(28):10311-6. doi: 10.1073/pnas.1409928111. Epub 2014 Jun 23. Proc Natl Acad Sci U S A. 2014. PMID: 24958881 Free PMC article.
-
Defining the antigenic diversity of Plasmodium falciparum apical membrane antigen 1 and the requirements for a multi-allele vaccine against malaria.PLoS One. 2012;7(12):e51023. doi: 10.1371/journal.pone.0051023. Epub 2012 Dec 5. PLoS One. 2012. PMID: 23227229 Free PMC article.
-
Apical membrane antigen 1 as an anti-malarial drug target.Curr Top Med Chem. 2011;11(16):2039-47. doi: 10.2174/156802611796575885. Curr Top Med Chem. 2011. PMID: 21619512 Review.
-
Apical membrane antigen 1: a malaria vaccine candidate in review.Trends Parasitol. 2008 Feb;24(2):74-84. doi: 10.1016/j.pt.2007.12.002. Epub 2008 Jan 15. Trends Parasitol. 2008. PMID: 18226584 Review.
References
-
- World Health Organization. World Malaria Report 2022 (WHO, 2022).
MeSH terms
Substances
LinkOut - more resources
Full Text Sources