Visualizing chaperonin function in situ by cryo-electron tomography
- PMID: 39169181
- PMCID: PMC11390479
- DOI: 10.1038/s41586-024-07843-w
Visualizing chaperonin function in situ by cryo-electron tomography
Abstract
Chaperonins are large barrel-shaped complexes that mediate ATP-dependent protein folding1-3. The bacterial chaperonin GroEL forms juxtaposed rings that bind unfolded protein and the lid-shaped cofactor GroES at their apertures. In vitro analyses of the chaperonin reaction have shown that substrate protein folds, unimpaired by aggregation, while transiently encapsulated in the GroEL central cavity by GroES4-6. To determine the functional stoichiometry of GroEL, GroES and client protein in situ, here we visualized chaperonin complexes in their natural cellular environment using cryo-electron tomography. We find that, under various growth conditions, around 55-70% of GroEL binds GroES asymmetrically on one ring, with the remainder populating symmetrical complexes. Bound substrate protein is detected on the free ring of the asymmetrical complex, defining the substrate acceptor state. In situ analysis of GroEL-GroES chambers, validated by high-resolution structures obtained in vitro, showed the presence of encapsulated substrate protein in a folded state before release into the cytosol. Based on a comprehensive quantification and conformational analysis of chaperonin complexes, we propose a GroEL-GroES reaction cycle that consists of linked asymmetrical and symmetrical subreactions mediating protein folding. Our findings illuminate the native conformational and functional chaperonin cycle directly within cells.
© 2024. The Author(s).
Conflict of interest statement
The authors declare no competing interests.
Figures
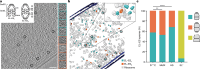
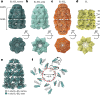
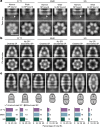
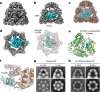
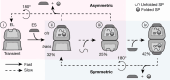
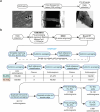
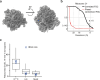
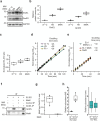
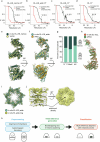
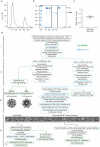
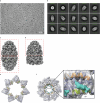
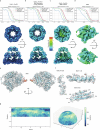
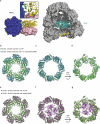
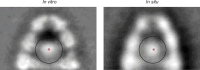
Similar articles
-
TEM and STEM-EDS evaluation of metal nanoparticle encapsulation in GroEL/GroES complexes according to the reaction mechanism of chaperonin.Microscopy (Oxf). 2021 Jun 6;70(3):289-296. doi: 10.1093/jmicro/dfaa064. Microscopy (Oxf). 2021. PMID: 33173948
-
Functional significance of symmetrical versus asymmetrical GroEL-GroES chaperonin complexes.Science. 1995 Aug 11;269(5225):832-6. doi: 10.1126/science.7638600. Science. 1995. PMID: 7638600
-
Chaperonin-Assisted Protein Folding: Relative Population of Asymmetric and Symmetric GroEL:GroES Complexes.J Mol Biol. 2015 Jun 19;427(12):2244-55. doi: 10.1016/j.jmb.2015.04.009. Epub 2015 Apr 23. J Mol Biol. 2015. PMID: 25912285
-
GroEL and the GroEL-GroES Complex.Subcell Biochem. 2017;83:483-504. doi: 10.1007/978-3-319-46503-6_17. Subcell Biochem. 2017. PMID: 28271487 Review.
-
Reaction Cycle of Chaperonin GroEL via Symmetric "Football" Intermediate.J Mol Biol. 2015 Sep 11;427(18):2912-8. doi: 10.1016/j.jmb.2015.04.007. Epub 2015 Apr 18. J Mol Biol. 2015. PMID: 25900372 Review.
Cited by
-
In-Situ Structure and Topography of AMPA Receptor Scaffolding Complexes Visualized by CryoET.bioRxiv [Preprint]. 2024 Oct 22:2024.10.19.619226. doi: 10.1101/2024.10.19.619226. bioRxiv. 2024. PMID: 39464045 Free PMC article. Preprint.
References
MeSH terms
Substances
LinkOut - more resources
Full Text Sources
Research Materials