In-cell bioluminescence resonance energy transfer (BRET)-based assay uncovers ceritinib and CA-074 as SARS-CoV-2 papain-like protease inhibitors
- PMID: 39163165
- PMCID: PMC11338211
- DOI: 10.1080/14756366.2024.2387417
In-cell bioluminescence resonance energy transfer (BRET)-based assay uncovers ceritinib and CA-074 as SARS-CoV-2 papain-like protease inhibitors
Abstract
Papain-like protease (PLpro) is an attractive anti-coronavirus target. The development of PLpro inhibitors, however, is hampered by the limitations of the existing PLpro assay and the scarcity of validated active compounds. We developed a novel in-cell PLpro assay based on BRET and used it to evaluate and discover SARS-CoV-2 PLpro inhibitors. The developed assay demonstrated remarkable sensitivity for detecting the reduction of intracellular PLpro activity while presenting high reliability and performance for inhibitor evaluation and high-throughput screening. Using this assay, three protease inhibitors were identified as novel PLpro inhibitors that are structurally disparate from those previously known. Subsequent enzymatic assays and ligand-protein interaction analysis based on molecular docking revealed that ceritinib directly inhibited PLpro, showing high geometric complementarity with the substrate-binding pocket in PLpro, whereas CA-074 methyl ester underwent intracellular hydrolysis, exposing a free carboxyhydroxyl group essential for hydrogen bonding with G266 in the BL2 groove, resulting in PLpro inhibition.
Keywords: SARS-CoV-2; bioluminescence resonance energy transfer (BRET); high-throughput screening (HTS); papain-like protease (PLpro); protease inhibitors.
Conflict of interest statement
No potential conflict of interest was reported by the author(s).
Figures
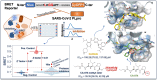
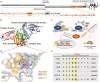
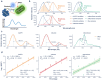
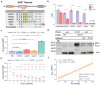
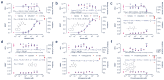
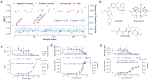
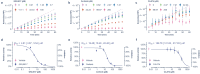
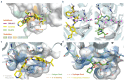
Similar articles
-
Development of potent and selective inhibitors targeting the papain-like protease of SARS-CoV-2.Cell Chem Biol. 2021 Jun 17;28(6):855-865.e9. doi: 10.1016/j.chembiol.2021.04.020. Epub 2021 Apr 27. Cell Chem Biol. 2021. PMID: 33979649 Free PMC article.
-
A novel robust inhibitor of papain-like protease (PLpro) as a COVID-19 drug.J Biomol Struct Dyn. 2024 Aug;42(13):6863-6870. doi: 10.1080/07391102.2023.2245474. Epub 2023 Aug 14. J Biomol Struct Dyn. 2024. PMID: 37578047
-
Non-Covalent Inhibitors of SARS-CoV-2 Papain-Like Protease (PLpro): In Vitro and In Vivo Antiviral Activity.J Med Chem. 2024 Aug 22;67(16):13681-13702. doi: 10.1021/acs.jmedchem.4c00378. Epub 2024 Aug 5. J Med Chem. 2024. PMID: 39102360 Free PMC article.
-
Chalcone-amide, a privileged backbone for the design and development of selective SARS-CoV/SARS-CoV-2 papain-like protease inhibitors.Eur J Med Chem. 2022 Oct 5;240:114572. doi: 10.1016/j.ejmech.2022.114572. Epub 2022 Jul 3. Eur J Med Chem. 2022. PMID: 35797899 Free PMC article. Review.
-
Design of inhibitors of SARS-CoV-2 papain-like protease deriving from GRL0617: Structure-activity relationships.Bioorg Med Chem. 2024 Nov 1;113:117909. doi: 10.1016/j.bmc.2024.117909. Epub 2024 Sep 11. Bioorg Med Chem. 2024. PMID: 39288705 Review.
References
-
- Toussi SS, Hammond JL, Gerstenberger BS, Anderson AS.. Therapeutics for COVID-19. Nat Microbiol. 2023;8(5):771–786. - PubMed
MeSH terms
Substances
Grants and funding
LinkOut - more resources
Full Text Sources
Other Literature Sources
Miscellaneous