This is a preprint.
Dual-Action Kinase Inhibitors Influence p38α MAP Kinase Dephosphorylation
- PMID: 39149408
- PMCID: PMC11326130
- DOI: 10.1101/2024.05.15.594272
Dual-Action Kinase Inhibitors Influence p38α MAP Kinase Dephosphorylation
Update in
-
Dual-action kinase inhibitors influence p38α MAP kinase dephosphorylation.Proc Natl Acad Sci U S A. 2025 Jan 7;122(1):e2415150122. doi: 10.1073/pnas.2415150122. Epub 2024 Dec 31. Proc Natl Acad Sci U S A. 2025. PMID: 39739785
Abstract
Reversible protein phosphorylation directs essential cellular processes including cell division, cell growth, cell death, inflammation, and differentiation. Because protein phosphorylation drives diverse diseases, kinases and phosphatases have been targets for drug discovery, with some achieving remarkable clinical success. Most protein kinases are activated by phosphorylation of their activation loops, which shifts the conformational equilibrium of the kinase towards the active state. To turn off the kinase, protein phosphatases dephosphorylate these sites, but how the conformation of the dynamic activation loop contributes to dephosphorylation was not known. To answer this, we modulated the activation loop conformational equilibrium of human p38α ΜΑP kinase with existing kinase inhibitors that bind and stabilize specific inactive activation loop conformations. From this, we discovered three inhibitors that increase the rate of dephosphorylation of the activation loop phospho-threonine by the PPM serine/threonine phosphatase WIP1. Hence, these compounds are "dual-action" inhibitors that simultaneously block the active site and stimulate p38α dephosphorylation. Our X-ray crystal structures of phosphorylated p38α bound to the dual-action inhibitors reveal a shared flipped conformation of the activation loop with a fully accessible phospho-threonine. In contrast, our X-ray crystal structure of phosphorylated apo human p38α reveals a different activation loop conformation with an inaccessible phospho-threonine, thereby explaining the increased rate of dephosphorylation upon inhibitor binding. These findings reveal a conformational preference of phosphatases for their targets and suggest a new approach to achieving improved potency and specificity for therapeutic kinase inhibitors.
Conflict of interest statement
Competing interests N.B. and E.S. are the inventors on a pending patent on a new method for optimizing kinase inhibitors applied for by Brandeis University. D.K. is co-founder of Relay Therapeutics and MOMA Therapeutics. The remaining authors declare no competing interests.
Figures
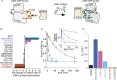
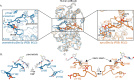
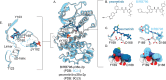
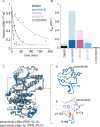
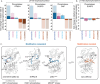
Similar articles
-
Dual-action kinase inhibitors influence p38α MAP kinase dephosphorylation.Proc Natl Acad Sci U S A. 2025 Jan 7;122(1):e2415150122. doi: 10.1073/pnas.2415150122. Epub 2024 Dec 31. Proc Natl Acad Sci U S A. 2025. PMID: 39739785
-
Depressing time: Waiting, melancholia, and the psychoanalytic practice of care.In: Kirtsoglou E, Simpson B, editors. The Time of Anthropology: Studies of Contemporary Chronopolitics. Abingdon: Routledge; 2020. Chapter 5. In: Kirtsoglou E, Simpson B, editors. The Time of Anthropology: Studies of Contemporary Chronopolitics. Abingdon: Routledge; 2020. Chapter 5. PMID: 36137063 Free Books & Documents. Review.
-
Using Experience Sampling Methodology to Capture Disclosure Opportunities for Autistic Adults.Autism Adulthood. 2023 Dec 1;5(4):389-400. doi: 10.1089/aut.2022.0090. Epub 2023 Dec 12. Autism Adulthood. 2023. PMID: 38116059 Free PMC article.
-
Dynamic Field Theory of Executive Function: Identifying Early Neurocognitive Markers.Monogr Soc Res Child Dev. 2024 Dec;89(3):7-109. doi: 10.1111/mono.12478. Monogr Soc Res Child Dev. 2024. PMID: 39628288 Free PMC article.
-
Trends in Surgical and Nonsurgical Aesthetic Procedures: A 14-Year Analysis of the International Society of Aesthetic Plastic Surgery-ISAPS.Aesthetic Plast Surg. 2024 Oct;48(20):4217-4227. doi: 10.1007/s00266-024-04260-2. Epub 2024 Aug 5. Aesthetic Plast Surg. 2024. PMID: 39103642 Review.
References
-
- Manning G., Whyte D. B., Martinez R., Hunter T. & Sudarsanam S. The Protein Kinase Complement of the Human Genome. Science 298, 1912–1934 (2002). - PubMed
-
- Huse M. & Kuriyan J. The conformational plasticity of protein kinases. Cell 109, 275–282 (2002). - PubMed
-
- Endicott J. A., Noble M. E. M. & Johnson L. N. The structural basis for control of eukaryotic protein kinases. Annu. Rev. Biochem. 81, 587–613 (2012). - PubMed
Publication types
Grants and funding
LinkOut - more resources
Full Text Sources