RiboScreenTM Technology Delivers a Ribosomal Target and a Small-Molecule Ligand for Ribosome Editing to Boost the Production Levels of Tropoelastin, the Monomeric Unit of Elastin
- PMID: 39125999
- PMCID: PMC11312584
- DOI: 10.3390/ijms25158430
RiboScreenTM Technology Delivers a Ribosomal Target and a Small-Molecule Ligand for Ribosome Editing to Boost the Production Levels of Tropoelastin, the Monomeric Unit of Elastin
Abstract
Elastin, a key structural protein essential for the elasticity of the skin and elastogenic tissues, degrades with age. Replenishing elastin holds promise for anti-aging cosmetics and the supplementation of elastic activities of the cardiovascular system. We employed RiboScreenTM, a technology for identifying molecules that enhance the production of specific proteins, to target the production of tropoelastin. We make use of RiboScreenTM in two crucial steps: first, to pinpoint a target ribosomal protein (TRP), which acts as a switch to increase the production of the protein of interest (POI), and second, to identify small molecules that activate this ribosomal protein switch. Using RiboScreenTM, we identified ribosomal protein L40, henceforth eL40, as a TRP switch to boost tropoelastin production. Drug discovery identified a small-molecule hit that binds to eL40. In-cell treatment demonstrated activity of the eL40 ligand and delivered increased tropoelastin production levels in a dose-dependent manner. Thus, we demonstrate that RiboScreenTM can successfully identify a small-molecule hit capable of selectively enhancing tropoelastin production. This compound has the potential to be developed for topical or systemic applications to promote skin rejuvenation and to supplement elastic functionality within the cardiovascular system.
Keywords: RiboScreenTM Technology; ageing of elastic tissues; customized protein expression; elastin; ribosomal protein eL40; small-molecule hit; tropoelastin expression.
Conflict of interest statement
H.B.-K., J.W.B., H.H. and J.K. are shareholders of KBHB Consult GmbH, Salzburg, Austria. J.V.H. and H.B.-K. are named as inventors in the patent family represented by WO24099952 A1 based on EP4368729, which is filed in the name of Merck Patent GmbH. The author Christin Rakers was employed by the company Merck Healthcare KGaA, Darmstadt, Germany. The author Daniel Kuhn was employed by the company Merck Healthcare KGaA, Darmstadt, Germany. The remaining authors declare that the research was conducted in the absence of any commercial or financial relationships that could be construed as a potential conflict of interest.
Figures
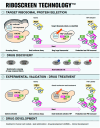

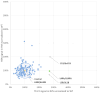
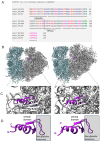
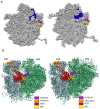
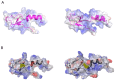
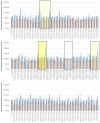
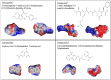
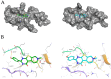
Similar articles
-
Sequence variants of human tropoelastin affecting assembly, structural characteristics and functional properties of polymeric elastin in health and disease.Matrix Biol. 2019 Nov;84:68-80. doi: 10.1016/j.matbio.2019.06.010. Epub 2019 Jun 26. Matrix Biol. 2019. PMID: 31254613 Review.
-
Tropoelastin.Int J Biochem Cell Biol. 2009 Mar;41(3):494-7. doi: 10.1016/j.biocel.2008.03.017. Epub 2008 Apr 1. Int J Biochem Cell Biol. 2009. PMID: 18468477 Review.
-
Elastin binding protein and FKBP65 modulate in vitro self-assembly of human tropoelastin.Biochemistry. 2013 Nov 5;52(44):7731-41. doi: 10.1021/bi400760f. Epub 2013 Oct 24. Biochemistry. 2013. PMID: 24106871
-
PKCε Increases Extracellular Elastin and Fibulin-5/DANCE in Dermal Fibroblasts.Cell Physiol Biochem. 2018;46(1):291-302. doi: 10.1159/000488430. Epub 2018 Mar 23. Cell Physiol Biochem. 2018. PMID: 29590645
-
Transglutaminase-Mediated Cross-Linking of Tropoelastin to Fibrillin Stabilises the Elastin Precursor Prior to Elastic Fibre Assembly.J Mol Biol. 2020 Oct 2;432(21):5736-5751. doi: 10.1016/j.jmb.2020.08.023. Epub 2020 Sep 6. J Mol Biol. 2020. PMID: 32898582 Free PMC article.
References
MeSH terms
Substances
Grants and funding
LinkOut - more resources
Full Text Sources