Pilot investigations into the mechanistic basis for adverse effects of glucocorticoids in dysferlinopathy
- PMID: 39123261
- PMCID: PMC11312411
- DOI: 10.1186/s13395-024-00350-6
Pilot investigations into the mechanistic basis for adverse effects of glucocorticoids in dysferlinopathy
Abstract
Background: Dysferlinopathies are a clinically heterogeneous group of muscular dystrophies caused by gene mutations resulting in deficiency of the membrane-associated protein dysferlin. They manifest post-growth and are characterised by muscle wasting (primarily in the limb and limb-gridle muscles), inflammation, and replacement of myofibres with adipose tissue. The precise pathomechanism for dysferlinopathy is currently unclear; as such there are no treatments currently available. Glucocorticoids (GCs) are widely used to reduce inflammation and treat muscular dystrophies, but when administered to patients with dysferlinopathy, they have unexpected adverse effects, with accelerated loss of muscle strength.
Methods: To investigate the mechanistic basis for the adverse effects of GCs in dysferlinopathy, the potent GC dexamethasone (Dex) was administered for 4-5 weeks (0.5-0.75 µg/mL in drinking water) to dysferlin-deficient BLA/J and normal wild-type (WT) male mice, sampled at 5 (Study 1) or 10 months (Study 2) of age. A wide range of analyses were conducted. Metabolism- and immune-related gene expression was assessed in psoas muscles at both ages and in quadriceps at 10 months of age. For the 10-month-old mice, quadriceps and psoas muscle histology was assessed. Additionally, we investigated the impact of Dex on the predominantly slow and fast-twitch soleus and extensor digitorum longus (EDL) muscles (respectively) in terms of contractile function, myofibre-type composition, and levels of proteins related to contractile function and metabolism, plus glycogen.
Results: At both ages, many complement-related genes were highly expressed in BLA/J muscles, and WT mice were generally more responsive to Dex than BLA/J. The effects of Dex on BLA/J mice included (i) increased expression of inflammasome-related genes in muscles (at 5 months) and (ii) exacerbated histopathology of quadriceps and psoas muscles at 10 months. A novel observation was pronounced staining for glycogen in many myofibres of the damaged quadriceps muscles, with large pale vacuolated myofibres, suggesting possible myofibre death by oncosis.
Conclusion: These pilot studies provide a new focus for further investigation into the adverse effects of GCs on dysferlinopathic muscles.
Keywords: Adipocytes; Complement; Dexamethasone; Dysferlin; Dysferlinopathy; Glucocorticoids; Glycogen; Inflammasome; Limb-girdle muscular dystrophy; Skeletal muscle.
© 2024. The Author(s).
Conflict of interest statement
The authors declare no competing interests.
Figures
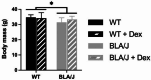
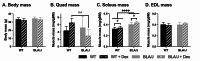
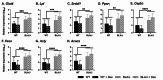
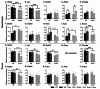
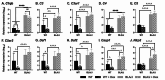
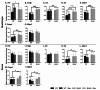
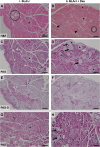
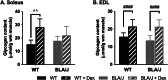
Similar articles
-
Dysferlin Deficiency Results in Myofiber-Type Specific Differences in Abundances of Calcium-Handling and Glycogen Metabolism Proteins.Int J Mol Sci. 2022 Dec 21;24(1):76. doi: 10.3390/ijms24010076. Int J Mol Sci. 2022. PMID: 36613515 Free PMC article.
-
Dysferlin-deficiency has greater impact on function of slow muscles, compared with fast, in aged BLAJ mice.PLoS One. 2019 Apr 10;14(4):e0214908. doi: 10.1371/journal.pone.0214908. eCollection 2019. PLoS One. 2019. PMID: 30970035 Free PMC article.
-
Lipid accumulation in dysferlin-deficient muscles.Am J Pathol. 2014 Jun;184(6):1668-76. doi: 10.1016/j.ajpath.2014.02.005. Epub 2014 Mar 29. Am J Pathol. 2014. PMID: 24685690 Clinical Trial.
-
Dysferlin function in skeletal muscle: Possible pathological mechanisms and therapeutical targets in dysferlinopathies.Exp Neurol. 2016 Sep;283(Pt A):246-54. doi: 10.1016/j.expneurol.2016.06.026. Epub 2016 Jun 25. Exp Neurol. 2016. PMID: 27349407 Review.
-
Muscular dystrophy in dysferlin-deficient mouse models.Neuromuscul Disord. 2013 May;23(5):377-87. doi: 10.1016/j.nmd.2013.02.004. Epub 2013 Mar 7. Neuromuscul Disord. 2013. PMID: 23473732 Review.
References
MeSH terms
Substances
Supplementary concepts
Grants and funding
LinkOut - more resources
Full Text Sources
Medical
Miscellaneous