Motor domain phosphorylation increases nucleotide exchange and turns MYO6 into a faster and stronger motor
- PMID: 39112473
- PMCID: PMC11306250
- DOI: 10.1038/s41467-024-49898-3
Motor domain phosphorylation increases nucleotide exchange and turns MYO6 into a faster and stronger motor
Abstract
Myosin motors perform many fundamental functions in eukaryotic cells by providing force generation, transport or tethering capacity. Motor activity control within the cell involves on/off switches, however, few examples are known of how myosins regulate speed or processivity and fine-tune their activity to a specific cellular task. Here, we describe a phosphorylation event for myosins of class VI (MYO6) in the motor domain, which accelerates its ATPase activity leading to a 4-fold increase in motor speed determined by actin-gliding assays, single molecule mechanics and stopped flow kinetics. We demonstrate that the serine/threonine kinase DYRK2 phosphorylates MYO6 at S267 in vitro. Single-molecule optical-tweezers studies at low load reveal that S267-phosphorylation results in faster nucleotide-exchange kinetics without change in the working stroke of the motor. The selective increase in stiffness of the acto-MYO6 complex when proceeding load-dependently into the nucleotide-free rigor state demonstrates that S267-phosphorylation turns MYO6 into a stronger motor. Finally, molecular dynamic simulations of the nucleotide-free motor reveal an alternative interaction network within insert-1 upon phosphorylation, suggesting a molecular mechanism, which regulates insert-1 positioning, turning the S267-phosphorylated MYO6 into a faster motor.
© 2024. Crown.
Conflict of interest statement
The authors declare no competing interests.
Figures
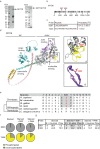
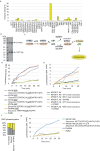
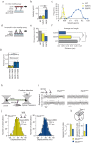
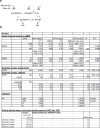
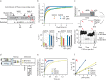
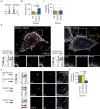
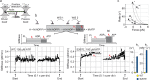
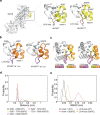
Similar articles
-
Plus-end directed myosins accelerate actin filament sliding by single-headed myosin VI.Cytoskeleton (Hoboken). 2012 Jan;69(1):59-69. doi: 10.1002/cm.21002. Epub 2012 Jan 9. Cytoskeleton (Hoboken). 2012. PMID: 22213699 Free PMC article.
-
Filopodia formation and endosome clustering induced by mutant plus-end-directed myosin VI.Proc Natl Acad Sci U S A. 2017 Feb 14;114(7):1595-1600. doi: 10.1073/pnas.1616941114. Epub 2017 Jan 31. Proc Natl Acad Sci U S A. 2017. PMID: 28143933 Free PMC article.
-
How myosin VI traps its off-state, is activated and dimerizes.Nat Commun. 2023 Oct 23;14(1):6732. doi: 10.1038/s41467-023-42376-2. Nat Commun. 2023. PMID: 37872146 Free PMC article.
-
The MYO6 interactome: selective motor-cargo complexes for diverse cellular processes.FEBS Lett. 2019 Jul;593(13):1494-1507. doi: 10.1002/1873-3468.13486. Epub 2019 Jul 3. FEBS Lett. 2019. PMID: 31206648 Review.
-
Diverse functions of myosin VI in spermiogenesis.Histochem Cell Biol. 2021 Mar;155(3):323-340. doi: 10.1007/s00418-020-01954-x. Epub 2021 Jan 2. Histochem Cell Biol. 2021. PMID: 33386429 Free PMC article. Review.
References
MeSH terms
Substances
Grants and funding
LinkOut - more resources
Full Text Sources