Tissue Engineered 3D Constructs for Volumetric Muscle Loss
- PMID: 39085677
- PMCID: PMC11329418
- DOI: 10.1007/s10439-024-03541-w
Tissue Engineered 3D Constructs for Volumetric Muscle Loss
Abstract
Severe injuries to skeletal muscles, including cases of volumetric muscle loss (VML), are linked to substantial tissue damage, resulting in functional impairment and lasting disability. While skeletal muscle can regenerate following minor damage, extensive tissue loss in VML disrupts the natural regenerative capacity of the affected muscle tissue. Existing clinical approaches for VML, such as soft-tissue reconstruction and advanced bracing methods, need to be revised to restore tissue function and are associated with limitations in tissue availability and donor-site complications. Advancements in tissue engineering (TE), particularly in scaffold design and the delivery of cells and growth factors, show promising potential for regenerating damaged skeletal muscle tissue and restoring function. This article provides a brief overview of the pathophysiology of VML and critiques the shortcomings of current treatments. The subsequent section focuses on the criteria for designing TE scaffolds, offering insights into various natural and synthetic biomaterials and cell types for effectively regenerating skeletal muscle. We also review multiple TE strategies involving both acellular and cellular scaffolds to encourage the development and maturation of muscle tissue and facilitate integration, vascularization, and innervation. Finally, the article explores technical challenges hindering successful translation into clinical applications.
Keywords: Biomaterials; Decellularization; Electrospinning; Hydrogels; Scaffolds; Skeletal muscle regeneration; Tissue engineering; Volumetric muscle loss.
© 2024. The Author(s).
Conflict of interest statement
The authors declare no conflicts of interest.
Figures
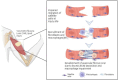
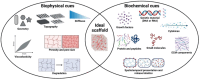
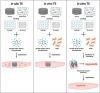
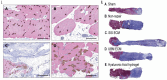
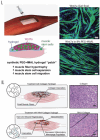
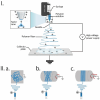
Similar articles
-
Cells, scaffolds, and bioactive factors: Engineering strategies for improving regeneration following volumetric muscle loss.Biomaterials. 2021 Nov;278:121173. doi: 10.1016/j.biomaterials.2021.121173. Epub 2021 Oct 1. Biomaterials. 2021. PMID: 34619561 Free PMC article. Review.
-
Skeletal Muscle Tissue Engineering: Biomaterials-Based Strategies for the Treatment of Volumetric Muscle Loss.Bioengineering (Basel). 2020 Jul 31;7(3):85. doi: 10.3390/bioengineering7030085. Bioengineering (Basel). 2020. PMID: 32751847 Free PMC article. Review.
-
Advances in electrospinning and 3D bioprinting strategies to enhance functional regeneration of skeletal muscle tissue.Biomater Adv. 2022 Nov;142:213135. doi: 10.1016/j.bioadv.2022.213135. Epub 2022 Sep 29. Biomater Adv. 2022. PMID: 36215745 Review.
-
Implantation of in vitro tissue engineered muscle repair constructs and bladder acellular matrices partially restore in vivo skeletal muscle function in a rat model of volumetric muscle loss injury.Tissue Eng Part A. 2014 Feb;20(3-4):705-15. doi: 10.1089/ten.TEA.2012.0761. Epub 2013 Dec 19. Tissue Eng Part A. 2014. PMID: 24066899 Free PMC article.
-
Vascularized and Innervated Skeletal Muscle Tissue Engineering.Adv Healthc Mater. 2020 Jan;9(1):e1900626. doi: 10.1002/adhm.201900626. Epub 2019 Oct 17. Adv Healthc Mater. 2020. PMID: 31622051 Free PMC article. Review.
References
-
- Sorensen, J. R., J. McFaline-Figueroa, and J. A. Call. Pathophysiology of volumetric muscle loss and targets for regenerative rehabilitation. In: Regenerative Rehabilitation: From Basic Science to the Clinic. Springer, 2022, pp. 177–225.
-
- Bartolacci, J. Scaffolds for skeletal muscle tissue engineering. In: Handbook of Tissue Engineering Scaffolds. 2019.
Publication types
MeSH terms
LinkOut - more resources
Full Text Sources