Deciphering the Intracellular Action of the Antimicrobial Peptide A11 via an In-Depth Analysis of Its Effect on the Global Proteome of Acinetobacter baumannii
- PMID: 39075773
- PMCID: PMC11320580
- DOI: 10.1021/acsinfecdis.4c00160
Deciphering the Intracellular Action of the Antimicrobial Peptide A11 via an In-Depth Analysis of Its Effect on the Global Proteome of Acinetobacter baumannii
Abstract
The potential antimicrobial activity and low propensity to induce the development of bacterial resistance have rendered antimicrobial peptides (AMPs) as novel and ideal candidate therapeutic agents for the treatment of infections caused by drug-resistant pathogenic bacteria. The targeting of bacterial membranes by AMPs has been typically considered their sole mode of action; however, increasing evidence supports the existence of multiple and complementary functions of AMPs that result in bacterial death. An in-depth characterization of their mechanism of action could facilitate further research and development of AMPs with higher potency. The current study employs biophysics and proteomics approaches to unveil the mechanisms underlying the antibacterial activity of A11, a potential candidate AMP, against Acinetobacter baumannii, a leading cause of hospital-acquired infections (HAIs) and consequently, a serious global threat. A11 peptide was found to induce membrane depolarization to a high extent, as revealed by flow cytometry and electron microscopy analyses. The prompt intracellular penetration of A11 peptide, observed using confocal microscopy, was found to occur concomitantly with a very low degree of membrane lysis, suggesting that its mode of action predominantly involves a nonlytic killing mechanism. Quantitative proteomics analysis employed for obtaining insights into the mechanisms underlying the antimicrobial activity of A11 peptide revealed that it disrupted energy metabolism, interfered with protein homeostasis, and inhibited fatty acid synthesis that is essential for cell membrane integrity; all these impacted the cellular functions of A. baumannii. A11 treatment also impacted signal transduction associated with the regulation of biofilm formation, hindered the stress response, and influenced DNA repair processes; these are all crucial survival mechanisms of A. baumannii. Additionally, robust antibacterial activity was exhibited by A11 peptide against multidrug-resistant (MDR) and extensively drug-resistant (XDR) clinical isolates of A. baumannii; moreover, A11 peptide exhibited synergy with levofloxacin and minocycline as well as low propensity for inducing resistance. Taken together, the findings emphasize the therapeutic potential of A11 peptide as an antibacterial agent against drug-resistant A. baumannii and underscore the need for further investigation.
Keywords: A11 peptide; Acinetobacter baumannii; antimicrobial peptides; intracellular actions; proteomic analysis; therapeutic potential.
Conflict of interest statement
The authors declare no competing financial interest.
Figures
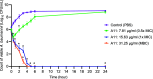
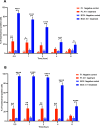
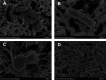
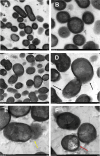
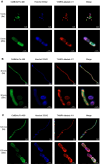
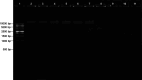
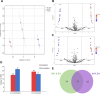
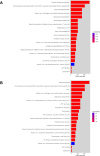
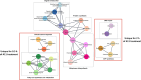
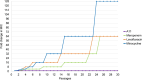
Similar articles
-
Unveiling the Self-assembly and Therapeutic Efficacy of Antimicrobial Peptides SA4 Against Multidrug-Resistant A. baumannii.Curr Microbiol. 2024 Oct 7;81(11):395. doi: 10.1007/s00284-024-03923-2. Curr Microbiol. 2024. PMID: 39375209
-
Antimicrobial and Anti-Inflammatory Activities of MAF-1-Derived Antimicrobial Peptide Mt6 and Its D-Enantiomer D-Mt6 against Acinetobacter baumannii by Targeting Cell Membranes and Lipopolysaccharide Interaction.Microbiol Spectr. 2022 Oct 26;10(5):e0131222. doi: 10.1128/spectrum.01312-22. Epub 2022 Oct 3. Microbiol Spectr. 2022. PMID: 36190276 Free PMC article.
-
Systemic Responses of Multidrug-Resistant Pseudomonas aeruginosa and Acinetobacter baumannii Following Exposure to the Antimicrobial Peptide Cathelicidin-BF Imply Multiple Intracellular Targets.Front Cell Infect Microbiol. 2017 Nov 7;7:466. doi: 10.3389/fcimb.2017.00466. eCollection 2017. Front Cell Infect Microbiol. 2017. PMID: 29164074 Free PMC article.
-
Antimicrobial peptides as a promising treatment option against Acinetobacter baumannii infections.Microb Pathog. 2020 Sep;146:104238. doi: 10.1016/j.micpath.2020.104238. Epub 2020 May 5. Microb Pathog. 2020. PMID: 32387392 Review.
-
Therapeutic Prospection of Animal Venoms-Derived Antimicrobial Peptides against Infections by Multidrug-Resistant Acinetobacter baumannii: A Systematic Review of Pre-Clinical Studies.Toxins (Basel). 2023 Apr 3;15(4):268. doi: 10.3390/toxins15040268. Toxins (Basel). 2023. PMID: 37104206 Free PMC article. Review.
Cited by
-
Origami of KR-12 Designed Antimicrobial Peptides and Their Potential Applications.Antibiotics (Basel). 2024 Aug 28;13(9):816. doi: 10.3390/antibiotics13090816. Antibiotics (Basel). 2024. PMID: 39334990 Free PMC article. Review.
References
-
- Zhang J.; Ouyang X.; Zhang F.; Li B.; Chang L.; Yang P.; Mao W.; Gou S.; Zhang Y.; Liu H.; Yao J.; Ni J. Structure-Activity Relationship Study of Antimicrobial Peptide PE2 Delivered Novel Linear Derivatives with Potential of Eradicating Biofilms and Low Incidence of Drug Resistance. J. Med. Chem. 2023, 66 (13), 8526–8544. 10.1021/acs.jmedchem.3c00181. - DOI - PubMed
-
- Khan H. A.; Baig F. K.; Mehboob R. Nosocomial infections: Epidemiology, prevention, control and surveillance. Asian Pac. J. Trop. Biomed. 2017, 7 (5), 478–482. 10.1016/j.apjtb.2017.01.019. - DOI
MeSH terms
Substances
LinkOut - more resources
Full Text Sources
Medical