The Mechanism of Action of L-Tyrosine Derivatives against Chikungunya Virus Infection In Vitro Depends on Structural Changes
- PMID: 39063216
- PMCID: PMC11277544
- DOI: 10.3390/ijms25147972
The Mechanism of Action of L-Tyrosine Derivatives against Chikungunya Virus Infection In Vitro Depends on Structural Changes
Abstract
Although the disease caused by chikungunya virus (CHIKV) is of great interest to public health organizations around the world, there are still no authorized antivirals for its treatment. Previously, dihalogenated anti-CHIKV compounds derived from L-tyrosine (dH-Y) were identified as being effective against in vitro infection by this virus, so the objective of this study was to determine the mechanisms of its antiviral action. Six dH-Y compounds (C1 to C6) dihalogenated with bromine or chlorine and modified in their amino groups were evaluated by different in vitro antiviral strategies and in silico tools. When the cells were exposed before infection, all compounds decreased the expression of viral proteins; only C4, C5 and C6 inhibited the genome; and C1, C2 and C3 inhibited infectious viral particles (IVPs). Furthermore, C1 and C3 reduce adhesion, while C2 and C3 reduce internalization, which could be related to the in silico interaction with the fusion peptide of the E1 viral protein. Only C3, C4, C5 and C6 inhibited IVPs when the cells were exposed after infection, and their effect occurred in late stages after viral translation and replication, such as assembly, and not during budding. In summary, the structural changes of these compounds determine their mechanism of action. Additionally, C3 was the only compound that inhibited CHIKV infection at different stages of the replicative cycle, making it a compound of interest for conversion as a potential drug.
Keywords: antiviral; chikungunya virus; computational biology; in vitro; mechanism of action; tyrosine.
Conflict of interest statement
The authors declare no conflict of interest.
Figures
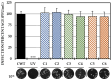
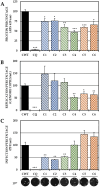
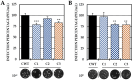
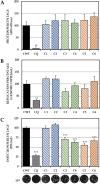
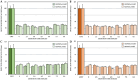
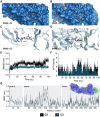
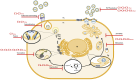
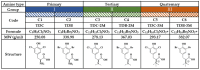
Similar articles
-
Serotonergic Drugs Inhibit Chikungunya Virus Infection at Different Stages of the Cell Entry Pathway.J Virol. 2020 Jun 16;94(13):e00274-20. doi: 10.1128/JVI.00274-20. Print 2020 Jun 16. J Virol. 2020. PMID: 32321803 Free PMC article.
-
In Vitro and In Silico Anti-Arboviral Activities of Dihalogenated Phenolic Derivates of L-Tyrosine.Molecules. 2021 Jun 5;26(11):3430. doi: 10.3390/molecules26113430. Molecules. 2021. PMID: 34198817 Free PMC article.
-
4'-Fluorouridine inhibits alphavirus replication and infection in vitro and in vivo.mBio. 2024 Jun 12;15(6):e0042024. doi: 10.1128/mbio.00420-24. Epub 2024 May 3. mBio. 2024. PMID: 38700353 Free PMC article.
-
Antivirals against the Chikungunya Virus.Viruses. 2021 Jul 5;13(7):1307. doi: 10.3390/v13071307. Viruses. 2021. PMID: 34372513 Free PMC article. Review.
-
Computer-Aided Structure Based Drug Design Approaches for the Discovery of New Anti-CHIKV Agents.Curr Comput Aided Drug Des. 2017 Nov 10;13(4):346-361. doi: 10.2174/1573409913666170309145308. Curr Comput Aided Drug Des. 2017. PMID: 28294048 Review.
References
-
- Ramirez R.M.G., Bohers C., Mousson L., Madec Y., Vazeille M., Piorkowski G., Moutailler S., Diaz F.J., Rua-Uribe G., Villar L.A., et al. Increased threat of urban arboviral diseases from Aedes aegypti mosquitoes in Colombia. IJID Reg. 2024;11:100360. doi: 10.1016/j.ijregi.2024.100360. - DOI - PMC - PubMed
-
- de Souza W.M., Fumagalli M.J., de Lima S.T.S., Parise P.L., Carvalho D.C.M., Hernandez C., de Jesus R., Delafiori J., Candido D.S., Carregari V.C., et al. Pathophysiology of chikungunya virus infection associated with fatal outcomes. Cell Host Microbe. 2024;32:606–622.e8. doi: 10.1016/j.chom.2024.02.011. - DOI - PMC - PubMed
-
- Ruiz-López F., González-Mazo A., Vélez-Mira A., Gómez G.F., Zuleta L., Uribe S., Vélez-Bernal I.D. Presencia de Aedes (Stegomyia) aegypti (Linnaeus, 1762) y su infección natural con el virus del dengue en alturas no registradas para Colombia. Biomédica. 2016;36:303–308. doi: 10.7705/biomedica.v36i2.3301. - DOI - PubMed
MeSH terms
Substances
LinkOut - more resources
Full Text Sources
Medical
Miscellaneous