Neurophysiological Oscillatory Mechanisms Underlying the Effect of Mirror Visual Feedback-Induced Illusion of Hand Movements on Nociception and Cortical Activation
- PMID: 39061436
- PMCID: PMC11274372
- DOI: 10.3390/brainsci14070696
Neurophysiological Oscillatory Mechanisms Underlying the Effect of Mirror Visual Feedback-Induced Illusion of Hand Movements on Nociception and Cortical Activation
Abstract
Mirror Visual Feedback (MVF)-induced illusion of hand movements produces beneficial effects in patients with chronic pain. However, neurophysiological mechanisms underlying these effects are poorly known. In this preliminary study, we test the novel hypothesis that such an MVF-induced movement illusion may exert its effects by changing the activity in midline cortical areas associated with pain processing. Electrical stimuli with individually fixed intensity were applied to the left hand of healthy adults to produce painful and non-painful sensations during unilateral right-hand movements with such an MVF illusion and right and bilateral hand movements without MVF. During these events, electroencephalographic (EEG) activity was recorded from 64 scalp electrodes. Event-related desynchronization (ERD) of EEG alpha rhythms (8-12 Hz) indexed the neurophysiological oscillatory mechanisms inducing cortical activation. Compared to the painful sensations, the non-painful sensations were specifically characterized by (1) lower alpha ERD estimated in the cortical midline, angular gyrus, and lateral parietal regions during the experimental condition with MVF and (2) higher alpha ERD estimated in the lateral prefrontal and parietal regions during the control conditions without MVF. These preliminary results suggest that the MVF-induced movement illusion may affect nociception and neurophysiological oscillatory mechanisms, reducing the activation in cortical limbic and default mode regions.
Keywords: Mirror Visual Feedback (MVF); alpha event-related de/synchronization (ERD/ERS); high-density electroencephalography (HD-EEG); pain; sensory–motor interaction; source analysis.
Conflict of interest statement
The authors declare no conflicts of interest.
Figures
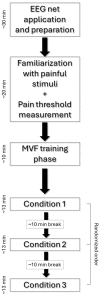
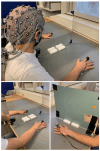
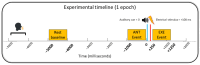
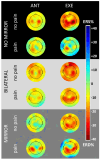
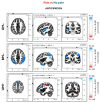
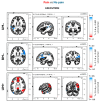
Similar articles
-
Cortical sources of electroencephalographic alpha rhythms related to the anticipation and experience of mirror visual feedback-induced illusion of finger movements.Psychophysiology. 2023 Jun;60(6):e14281. doi: 10.1111/psyp.14281. Epub 2023 Feb 28. Psychophysiology. 2023. PMID: 36852668
-
Mirror visual feedback during unilateral finger movements is related to the desynchronization of cortical electroencephalographic somatomotor alpha rhythms.Psychophysiology. 2022 Dec;59(12):e14116. doi: 10.1111/psyp.14116. Epub 2022 Jun 3. Psychophysiology. 2022. PMID: 35657095 Free PMC article.
-
Cortical Functional Domains Show Distinctive Oscillatory Dynamic in Bimanual and Mirror Visual Feedback Tasks.Front Comput Neurosci. 2019 May 9;13:30. doi: 10.3389/fncom.2019.00030. eCollection 2019. Front Comput Neurosci. 2019. PMID: 31143108 Free PMC article.
-
Reflections on mirror therapy: a systematic review of the effect of mirror visual feedback on the brain.Neurorehabil Neural Repair. 2015 May;29(4):349-61. doi: 10.1177/1545968314546134. Epub 2014 Aug 26. Neurorehabil Neural Repair. 2015. PMID: 25160567 Review.
-
Cortical EEG alpha rhythms reflect task-specific somatosensory and motor interactions in humans.Clin Neurophysiol. 2014 Oct;125(10):1936-45. doi: 10.1016/j.clinph.2014.04.021. Epub 2014 May 24. Clin Neurophysiol. 2014. PMID: 24929901 Review.
Cited by
-
Role of Immersive Virtual Reality in Motor Behaviour Decision-Making in Chronic Pain Patients.Brain Sci. 2023 Apr 5;13(4):617. doi: 10.3390/brainsci13040617. Brain Sci. 2023. PMID: 37190582 Free PMC article. Review.
References
-
- Ortiz-Catalan M., Guðmundsdóttir R.A., Kristoffersen M.B., Zepeda-Echavarria A., Caine-Winterberger K., Kulbacka-Ortiz K., Widehammar C., Eriksson K., Stockselius A., Ragnö C., et al. Phantom Motor Execution Facilitated by Machine Learning and Augmented Reality as Treatment for Phantom Limb Pain: A Single Group, Clinical Trial in Patients with Chronic Intractable Phantom Limb Pain. Lancet. 2016;388:2885–2894. doi: 10.1016/S0140-6736(16)31598-7. - DOI - PubMed
-
- Ding L., Wang X., Guo X., Chen S., Wang H., Cui X., Rong J., Jia J. Effects of Camera-Based Mirror Visual Feedback Therapy for Patients Who Had a Stroke and the Neural Mechanisms Involved: Protocol of a Multicentre Randomised Control Study. BMJ Open. 2019;9:e022828. doi: 10.1136/bmjopen-2018-022828. - DOI - PMC - PubMed
-
- Herrador Colmenero L., Perez Marmol J.M., Martí-García C., Querol Zaldivar M.D., Tapia Haro R.M., Castro Sánchez A.M., Aguilar-Ferrándiz M.E. Effectiveness of Mirror Therapy, Motor Imagery, and Virtual Feedback on Phantom Limb Pain Following Amputation: A Systematic Review. Prosthet. Orthot. Int. 2018;42:288–298. doi: 10.1177/0309364617740230. - DOI - PubMed
Grants and funding
LinkOut - more resources
Full Text Sources