Purinergic Ca2+ Signaling as a Novel Mechanism of Drug Tolerance in BRAF-Mutant Melanoma
- PMID: 39001489
- PMCID: PMC11240618
- DOI: 10.3390/cancers16132426
Purinergic Ca2+ Signaling as a Novel Mechanism of Drug Tolerance in BRAF-Mutant Melanoma
Abstract
Drug tolerance is a major cause of relapse after cancer treatment. Despite intensive efforts, its molecular basis remains poorly understood, hampering actionable intervention. We report a previously unrecognized signaling mechanism supporting drug tolerance in BRAF-mutant melanoma treated with BRAF inhibitors that could be of general relevance to other cancers. Its key features are cell-intrinsic intracellular Ca2+ signaling initiated by P2X7 receptors (purinergic ligand-gated cation channels) and an enhanced ability for these Ca2+ signals to reactivate ERK1/2 in the drug-tolerant state. Extracellular ATP, virtually ubiquitous in living systems, is the ligand that can initiate Ca2+ spikes via P2X7 channels. ATP is abundant in the tumor microenvironment and is released by dying cells, ironically implicating treatment-initiated cancer cell death as a source of trophic stimuli that leads to ERK reactivation and drug tolerance. Such a mechanism immediately offers an explanation of the inevitable relapse after BRAFi treatment in BRAF-mutant melanoma and points to actionable strategies to overcome it.
Keywords: ERK; P2X7; calcium signaling; non-genetic drug tolerance.
Conflict of interest statement
The authors have no conflicts of interest to report.
Figures
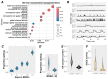
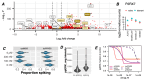
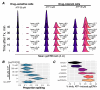
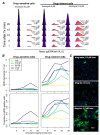
Update of
-
Purinergic Ca2+ signaling as a novel mechanism of drug tolerance in BRAF mutant melanoma.bioRxiv [Preprint]. 2024 Apr 1:2023.11.03.565532. doi: 10.1101/2023.11.03.565532. bioRxiv. 2024. Update in: Cancers (Basel). 2024 Jun 30;16(13):2426. doi: 10.3390/cancers16132426. PMID: 37961267 Free PMC article. Updated. Preprint.
Similar articles
-
Purinergic Ca2+ signaling as a novel mechanism of drug tolerance in BRAF mutant melanoma.bioRxiv [Preprint]. 2024 Apr 1:2023.11.03.565532. doi: 10.1101/2023.11.03.565532. bioRxiv. 2024. Update in: Cancers (Basel). 2024 Jun 30;16(13):2426. doi: 10.3390/cancers16132426. PMID: 37961267 Free PMC article. Updated. Preprint.
-
ER Translocation of the MAPK Pathway Drives Therapy Resistance in BRAF-Mutant Melanoma.Cancer Discov. 2019 Mar;9(3):396-415. doi: 10.1158/2159-8290.CD-18-0348. Epub 2018 Dec 18. Cancer Discov. 2019. PMID: 30563872 Free PMC article.
-
Kinetics of RTK activation determine ERK reactivation and resistance to dual BRAF/MEK inhibition in melanoma.Cell Rep. 2023 Jun 27;42(6):112570. doi: 10.1016/j.celrep.2023.112570. Epub 2023 May 29. Cell Rep. 2023. PMID: 37252843
-
Regulation and Role of Store-Operated Ca2+ Entry in Cellular Proliferation.In: Kozak JA, Putney JW Jr, editors. Calcium Entry Channels in Non-Excitable Cells. Boca Raton (FL): CRC Press/Taylor & Francis; 2018. Chapter 12. In: Kozak JA, Putney JW Jr, editors. Calcium Entry Channels in Non-Excitable Cells. Boca Raton (FL): CRC Press/Taylor & Francis; 2018. Chapter 12. PMID: 30299656 Free Books & Documents. Review.
-
The P2X7 Receptor in Inflammatory Diseases: Angel or Demon?Front Pharmacol. 2018 Feb 6;9:52. doi: 10.3389/fphar.2018.00052. eCollection 2018. Front Pharmacol. 2018. PMID: 29467654 Free PMC article. Review.
References
-
- Marin-Bejar O., Rogiers A., Dewaele M., Femel J., Karras P., Pozniak J., Bervoets G., Van Raemdonck N., Pedri D., Swings T., et al. Evolutionary Predictability of Genetic versus Nongenetic Resistance to Anticancer Drugs in Melanoma. Cancer Cell. 2021;39:1135–1149.e8. doi: 10.1016/j.ccell.2021.05.015. - DOI - PubMed
Grants and funding
LinkOut - more resources
Full Text Sources
Research Materials
Miscellaneous