Single-molecule characterization of SV40 replisome and novel factors: human FPC and Mcm10
- PMID: 38967018
- PMCID: PMC11347169
- DOI: 10.1093/nar/gkae565
Single-molecule characterization of SV40 replisome and novel factors: human FPC and Mcm10
Abstract
The simian virus 40 (SV40) replisome only encodes for its helicase; large T-antigen (L-Tag), while relying on the host for the remaining proteins, making it an intriguing model system. Despite being one of the earliest reconstituted eukaryotic systems, the interactions coordinating its activities and the identification of new factors remain largely unexplored. Herein, we in vitro reconstituted the SV40 replisome activities at the single-molecule level, including DNA unwinding by L-Tag and the single-stranded DNA-binding protein Replication Protein A (RPA), primer extension by DNA polymerase δ, and their concerted leading-strand synthesis. We show that RPA stimulates the processivity of L-Tag without altering its rate and that DNA polymerase δ forms a stable complex with L-Tag during leading-strand synthesis. Furthermore, similar to human and budding yeast Cdc45-MCM-GINS helicase, L-Tag uses the fork protection complex (FPC) and the mini-chromosome maintenance protein 10 (Mcm10) during synthesis. Hereby, we demonstrate that FPC increases this rate, and both FPC and Mcm10 increase the processivity by stabilizing stalled replisomes and increasing their chances of restarting synthesis. The detailed kinetics and novel factors of the SV40 replisome establish it as a closer mimic of the host replisome and expand its application as a model replication system.
© The Author(s) 2024. Published by Oxford University Press on behalf of Nucleic Acids Research.
Figures
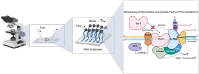
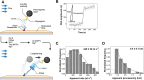
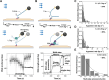
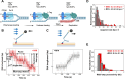
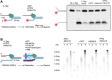
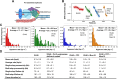
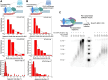
Similar articles
-
Functional interactions between SV40 T antigen and other replication proteins at the replication fork.J Biol Chem. 1993 May 25;268(15):11008-17. J Biol Chem. 1993. PMID: 8098707
-
Single-molecule visualization of Saccharomyces cerevisiae leading-strand synthesis reveals dynamic interaction between MTC and the replisome.Proc Natl Acad Sci U S A. 2017 Oct 3;114(40):10630-10635. doi: 10.1073/pnas.1711291114. Epub 2017 Sep 18. Proc Natl Acad Sci U S A. 2017. PMID: 28923950 Free PMC article.
-
An interaction between replication protein A and SV40 T antigen appears essential for primosome assembly during SV40 DNA replication.J Biol Chem. 1993 Feb 15;268(5):3389-95. J Biol Chem. 1993. PMID: 8381428
-
Eukaryotic DNA Replication Fork.Annu Rev Biochem. 2017 Jun 20;86:417-438. doi: 10.1146/annurev-biochem-061516-044709. Epub 2017 Mar 1. Annu Rev Biochem. 2017. PMID: 28301743 Free PMC article. Review.
-
Fine-tuning of the replisome: Mcm10 regulates fork progression and regression.Cell Cycle. 2019 May;18(10):1047-1055. doi: 10.1080/15384101.2019.1609833. Epub 2019 May 5. Cell Cycle. 2019. PMID: 31014174 Free PMC article. Review.
References
MeSH terms
Substances
Grants and funding
LinkOut - more resources
Full Text Sources
Miscellaneous