Mammalian START-like phosphatidylinositol transfer proteins - Physiological perspectives and roles in cancer biology
- PMID: 38945251
- PMCID: PMC11533902
- DOI: 10.1016/j.bbalip.2024.159529
Mammalian START-like phosphatidylinositol transfer proteins - Physiological perspectives and roles in cancer biology
Abstract
PtdIns and its phosphorylated derivatives, the phosphoinositides, are the biochemical components of a major pathway of intracellular signaling in all eukaryotic cells. These lipids are few in terms of cohort of unique positional isomers, and are quantitatively minor species of the bulk cellular lipidome. Nevertheless, phosphoinositides regulate an impressively diverse set of biological processes. It is from that perspective that perturbations in phosphoinositide-dependent signaling pathways are increasingly being recognized as causal foundations of many human diseases - including cancer. Although phosphatidylinositol transfer proteins (PITPs) are not enzymes, these proteins are physiologically significant regulators of phosphoinositide signaling. As such, PITPs are conserved throughout the eukaryotic kingdom. Their biological importance notwithstanding, PITPs remain understudied. Herein, we review current information regarding PITP biology primarily focusing on how derangements in PITP function disrupt key signaling/developmental pathways and are associated with a growing list of pathologies in mammals.
Keywords: Lipid signaling; Mammalian disease; Phosphatidylinositol transfer proteins; Phosphoinositides.
Copyright © 2024. Published by Elsevier B.V.
Conflict of interest statement
Declaration of competing interest Vytas A. Bankaitis reports financial support was provided by National Institutes of Health. Vytas A. Bankaitis reports financial support was provided by Robert A. Welch Foundation. If there are other authors, they declare that they have no known competing financial interests or personal relationships that could have appeared to influence the work reported in this paper.
Figures
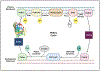
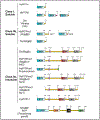
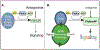
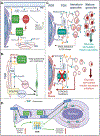
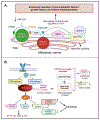
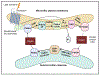
Similar articles
-
The interface between phosphatidylinositol transfer protein function and phosphoinositide signaling in higher eukaryotes.J Lipid Res. 2019 Feb;60(2):242-268. doi: 10.1194/jlr.R089730. Epub 2018 Nov 30. J Lipid Res. 2019. PMID: 30504233 Free PMC article. Review.
-
Devising powerful genetics, biochemical and structural tools in the functional analysis of phosphatidylinositol transfer proteins (PITPs) across diverse species.Methods Cell Biol. 2012;108:249-302. doi: 10.1016/B978-0-12-386487-1.00013-4. Methods Cell Biol. 2012. PMID: 22325607
-
Sec14-like phosphatidylinositol transfer proteins and the biological landscape of phosphoinositide signaling in plants.Biochim Biophys Acta. 2016 Sep;1861(9 Pt B):1352-1364. doi: 10.1016/j.bbalip.2016.03.027. Epub 2016 Mar 31. Biochim Biophys Acta. 2016. PMID: 27038688 Free PMC article. Review.
-
Dynamics and energetics of the mammalian phosphatidylinositol transfer protein phospholipid exchange cycle.J Biol Chem. 2017 Sep 1;292(35):14438-14455. doi: 10.1074/jbc.M117.791467. Epub 2017 Jul 17. J Biol Chem. 2017. PMID: 28718450 Free PMC article.
-
Phosphatidylinositol transfer proteins and instructive regulation of lipid kinase biology.Biochim Biophys Acta. 2015 Jun;1851(6):724-35. doi: 10.1016/j.bbalip.2014.12.011. Epub 2015 Jan 12. Biochim Biophys Acta. 2015. PMID: 25592381 Free PMC article. Review.
References
Publication types
MeSH terms
Substances
Grants and funding
LinkOut - more resources
Full Text Sources
Research Materials