Centrosome-organized plasma membrane infoldings linked to growth of a cortical actin domain
- PMID: 38935075
- PMCID: PMC11215285
- DOI: 10.1083/jcb.202403115
Centrosome-organized plasma membrane infoldings linked to growth of a cortical actin domain
Abstract
Regulated cell shape change requires the induction of cortical cytoskeletal domains. Often, local changes to plasma membrane (PM) topography are involved. Centrosomes organize cortical domains and can affect PM topography by locally pulling the PM inward. Are these centrosome effects coupled? At the syncytial Drosophila embryo cortex, centrosome-induced actin caps grow into dome-like compartments for mitoses. We found the nascent cap to be a collection of PM folds and tubules formed over the astral centrosomal MT array. The localized infoldings require centrosome and dynein activities, and myosin-based surface tension prevents them elsewhere. Centrosome-engaged PM infoldings become specifically enriched with an Arp2/3 induction pathway. Arp2/3 actin network growth between the infoldings counterbalances centrosomal pulling forces and disperses the folds for actin cap expansion. Abnormal domain topography with either centrosome or Arp2/3 disruption correlates with decreased exocytic vesicle association. Together, our data implicate centrosome-organized PM infoldings in coordinating Arp2/3 network growth and exocytosis for cortical domain assembly.
© 2024 Tam and Harris.
Conflict of interest statement
Disclosures: The authors declare no competing interests exist.
Figures
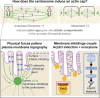
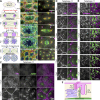
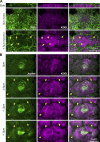
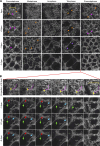
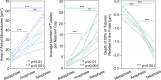
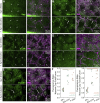
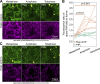
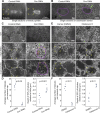
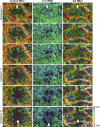
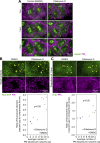
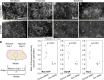
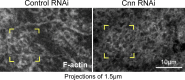
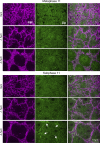
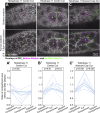
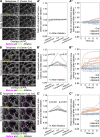
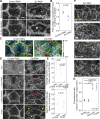
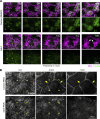
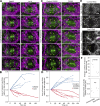
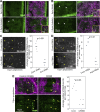
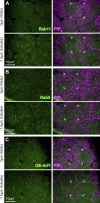
Similar articles
-
Depressing time: Waiting, melancholia, and the psychoanalytic practice of care.In: Kirtsoglou E, Simpson B, editors. The Time of Anthropology: Studies of Contemporary Chronopolitics. Abingdon: Routledge; 2020. Chapter 5. In: Kirtsoglou E, Simpson B, editors. The Time of Anthropology: Studies of Contemporary Chronopolitics. Abingdon: Routledge; 2020. Chapter 5. PMID: 36137063 Free Books & Documents. Review.
-
Functional analysis of centrosomal kinase substrates in Drosophila melanogaster reveals a new function of the nuclear envelope component otefin in cell cycle progression.Mol Cell Biol. 2012 Sep;32(17):3554-69. doi: 10.1128/MCB.00814-12. Epub 2012 Jul 2. Mol Cell Biol. 2012. PMID: 22751930 Free PMC article.
-
Evolutionary diversification reveals distinct somatic versus germline cytoskeletal functions of the Arp2 branched actin nucleator protein.Curr Biol. 2023 Dec 18;33(24):5326-5339.e7. doi: 10.1016/j.cub.2023.10.055. Epub 2023 Nov 16. Curr Biol. 2023. PMID: 37977138 Free PMC article.
-
"I've Spent My Whole Life Striving to Be Normal": Internalized Stigma and Perceived Impact of Diagnosis in Autistic Adults.Autism Adulthood. 2023 Dec 1;5(4):423-436. doi: 10.1089/aut.2022.0066. Epub 2023 Dec 12. Autism Adulthood. 2023. PMID: 38116050 Free PMC article.
-
The effectiveness of abstinence-based and harm reduction-based interventions in reducing problematic substance use in adults who are experiencing homelessness in high income countries: A systematic review and meta-analysis: A systematic review.Campbell Syst Rev. 2024 Apr 21;20(2):e1396. doi: 10.1002/cl2.1396. eCollection 2024 Jun. Campbell Syst Rev. 2024. PMID: 38645303 Free PMC article. Review.
References
MeSH terms
Substances
Grants and funding
LinkOut - more resources
Full Text Sources
Molecular Biology Databases
Miscellaneous