Precision in Action: The Role of Clustered Regularly Interspaced Short Palindromic Repeats/Cas in Gene Therapies
- PMID: 38932365
- PMCID: PMC11209408
- DOI: 10.3390/vaccines12060636
Precision in Action: The Role of Clustered Regularly Interspaced Short Palindromic Repeats/Cas in Gene Therapies
Abstract
Clustered Regularly Interspaced Short Palindromic Repeat (CRISPR)-associated enzyme-CAS holds great promise for treating many uncured human diseases and illnesses by precisely correcting harmful point mutations and disrupting disease-causing genes. The recent Food and Drug Association (FDA) approval of the first CRISPR-based gene therapy for sickle cell anemia marks the beginning of a new era in gene editing. However, delivering CRISPR specifically into diseased cells in vivo is a significant challenge and an area of intense research. The identification of new CRISPR/Cas variants, particularly ultra-compact CAS systems with robust gene editing activities, paves the way for the low-capacity delivery vectors to be used in gene therapies. CRISPR/Cas technology has evolved beyond editing DNA to cover a wide spectrum of functionalities, including RNA targeting, disease diagnosis, transcriptional/epigenetic regulation, chromatin imaging, high-throughput screening, and new disease modeling. CRISPR/Cas can be used to engineer B-cells to produce potent antibodies for more effective vaccines and enhance CAR T-cells for the more precise and efficient targeting of tumor cells. However, CRISPR/Cas technology has challenges, including off-target effects, toxicity, immune responses, and inadequate tissue-specific delivery. Overcoming these challenges necessitates the development of a more effective and specific CRISPR/Cas delivery system. This entails strategically utilizing specific gRNAs in conjunction with robust CRISPR/Cas variants to mitigate off-target effects. This review seeks to delve into the intricacies of the CRISPR/Cas mechanism, explore progress in gene therapies, evaluate gene delivery systems, highlight limitations, outline necessary precautions, and scrutinize the ethical considerations associated with its application.
Keywords: CRISPR/Cas; cancer; gene delivery; gene therapy; genetic disease; genome editing; infection; viral vectors.
Conflict of interest statement
The authors declare no conflicts of interest.
Figures
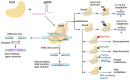
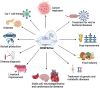
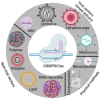
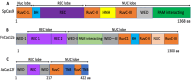
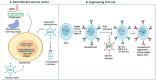
Similar articles
-
CRISPR/Cas: a Nobel Prize award-winning precise genome editing technology for gene therapy and crop improvement.J Zhejiang Univ Sci B. 2021 Apr 15;22(4):253-284. doi: 10.1631/jzus.B2100009. J Zhejiang Univ Sci B. 2021. PMID: 33835761 Free PMC article. Review.
-
CRISPR/Cas gene therapy.J Cell Physiol. 2021 Apr;236(4):2459-2481. doi: 10.1002/jcp.30064. Epub 2020 Sep 22. J Cell Physiol. 2021. PMID: 32959897 Review.
-
Applications of Clustered Regularly Interspaced Short Palindromic Repeats (CRISPR) as a Genetic Scalpel for the Treatment of Cancer: A Translational Narrative Review.Cureus. 2023 Dec 6;15(12):e50031. doi: 10.7759/cureus.50031. eCollection 2023 Dec. Cureus. 2023. PMID: 38186450 Free PMC article. Review.
-
CRISPR/Cas: Advances, Limitations, and Applications for Precision Cancer Research.Front Med (Lausanne). 2021 Mar 3;8:649896. doi: 10.3389/fmed.2021.649896. eCollection 2021. Front Med (Lausanne). 2021. PMID: 33748164 Free PMC article. Review.
-
Applications and challenges of CRISPR-Cas gene-editing to disease treatment in clinics.Precis Clin Med. 2021 Jul 10;4(3):179-191. doi: 10.1093/pcmedi/pbab014. eCollection 2021 Sep. Precis Clin Med. 2021. PMID: 34541453 Free PMC article. Review.
References
-
- Ishino Y., Shinagawa H., Makino K., Amemura M., Nakata A. Nucleotide sequence of the iap gene, responsible for alkaline phosphatase isozyme conversion in Escherichia coli, and identification of the gene product. J. Bacteriol. 1987;169:5429–5433. doi: 10.1128/jb.169.12.5429-5433.1987. - DOI - PMC - PubMed
Publication types
Grants and funding
LinkOut - more resources
Full Text Sources