Archaeosomes for Oral Drug Delivery: From Continuous Microfluidics Production to Powdered Formulations
- PMID: 38931818
- PMCID: PMC11206520
- DOI: 10.3390/pharmaceutics16060694
Archaeosomes for Oral Drug Delivery: From Continuous Microfluidics Production to Powdered Formulations
Abstract
Archaeosomes were manufactured from natural archaeal lipids by a microfluidics-assisted single-step production method utilizing a mixture of di- and tetraether lipids extracted from Sulfolobus acidocaldarius. The primary aim of this study was to investigate the exceptional stability of archaeosomes as potential carriers for oral drug delivery, with a focus on powdered formulations. The archaeosomes were negatively charged with a size of approximately 100 nm and a low polydispersity index. To assess their suitability for oral delivery, the archaeosomes were loaded with two model drugs: calcein, a fluorescent compound, and insulin, a peptide hormone. The archaeosomes demonstrated high stability in simulated intestinal fluids, with only 5% of the encapsulated compounds being released after 24 h, regardless of the presence of degrading enzymes or extremely acidic pH values such as those found in the stomach. In a co-culture cell model system mimicking the intestinal barrier, the archaeosomes showed strong adhesion to the cell membranes, facilitating a slow release of contents. The archaeosomes were loaded with insulin in a single-step procedure achieving an encapsulation efficiency of approximately 35%. These particles have been exposed to extreme manufacturing temperatures during freeze-drying and spray-drying processes, demonstrating remarkable resilience under these harsh conditions. The fabrication of stable dry powder formulations of archaeosomes represents a promising advancement toward the development of solid dosage forms for oral delivery of biological drugs.
Keywords: archaeal lipids; archaeosomes; dry powder formulation; insulin; oral drug delivery; solid dosage form.
Conflict of interest statement
The co-authors Christina Horn and Julian Quehenberger are currently employed by the company NovoArc GmbH, Vienna, Austria. The remaining authors declare that the research was conducted in the absence of any commercial or financial relationships that could be construed as a potential conflict of interest.
Figures
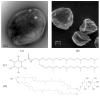
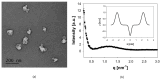
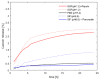
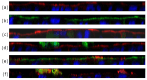
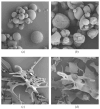
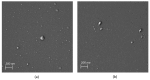
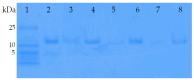
Similar articles
-
Polar Lipid Fraction E from Sulfolobus acidocaldarius and Dipalmitoylphosphatidylcholine Can Form Stable yet Thermo-Sensitive Tetraether/Diester Hybrid Archaeosomes with Controlled Release Capability.Int J Mol Sci. 2020 Nov 9;21(21):8388. doi: 10.3390/ijms21218388. Int J Mol Sci. 2020. PMID: 33182284 Free PMC article.
-
Investigation of archaeosomes as carriers for oral delivery of peptides.Biochem Biophys Res Commun. 2010 Apr 2;394(2):412-7. doi: 10.1016/j.bbrc.2010.03.041. Epub 2010 Mar 10. Biochem Biophys Res Commun. 2010. PMID: 20226174
-
Preparation and Characterization of Stealth Archaeosomes Based on a Synthetic PEGylated Archaeal Tetraether Lipid.J Drug Deliv. 2011;2011:396068. doi: 10.1155/2011/396068. Epub 2011 Mar 21. J Drug Deliv. 2011. PMID: 21603209 Free PMC article.
-
New generation of liposomes called archaeosomes based on natural or synthetic archaeal lipids as innovative formulations for drug delivery.Recent Pat Drug Deliv Formul. 2009 Nov;3(3):206-20. doi: 10.2174/187221109789105630. Recent Pat Drug Deliv Formul. 2009. PMID: 19534669 Review.
-
Archaeosomes: New Generation of Liposomes Based on Archaeal Lipids for Drug Delivery and Biomedical Applications.ACS Omega. 2022 Dec 28;8(1):1-9. doi: 10.1021/acsomega.2c06034. eCollection 2023 Jan 10. ACS Omega. 2022. PMID: 36643444 Free PMC article. Review.
References
Grants and funding
LinkOut - more resources
Full Text Sources