Advanced gene nanocarriers/scaffolds in nonviral-mediated delivery system for tissue regeneration and repair
- PMID: 38926780
- PMCID: PMC11200991
- DOI: 10.1186/s12951-024-02580-8
Advanced gene nanocarriers/scaffolds in nonviral-mediated delivery system for tissue regeneration and repair
Erratum in
-
Correction: Advanced gene nanocarriers/scaffolds in nonviral-mediated delivery system for tissue regeneration and repair.J Nanobiotechnology. 2024 Aug 29;22(1):516. doi: 10.1186/s12951-024-02769-x. J Nanobiotechnology. 2024. PMID: 39198850 Free PMC article. No abstract available.
Abstract
Tissue regeneration technology has been rapidly developed and widely applied in tissue engineering and repair. Compared with traditional approaches like surgical treatment, the rising gene therapy is able to have a durable effect on tissue regeneration, such as impaired bone regeneration, articular cartilage repair and cancer-resected tissue repair. Gene therapy can also facilitate the production of in situ therapeutic factors, thus minimizing the diffusion or loss of gene complexes and enabling spatiotemporally controlled release of gene products for tissue regeneration. Among different gene delivery vectors and supportive gene-activated matrices, advanced gene/drug nanocarriers attract exceptional attraction due to their tunable physiochemical properties, as well as excellent adaptive performance in gene therapy for tissue regeneration, such as bone, cartilage, blood vessel, nerve and cancer-resected tissue repair. This paper reviews the recent advances on nonviral-mediated gene delivery systems with an emphasis on the important role of advanced nanocarriers in gene therapy and tissue regeneration.
Keywords: Advanced gene/Drug nanocarriers; Cancer-resected tissue repair; Gene therapy; Nonviral-mediated delivery system; Tissue regeneration.
© 2024. The Author(s).
Conflict of interest statement
The authors declare no competing interests.
Figures
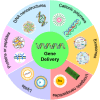
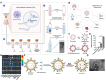
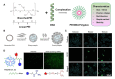
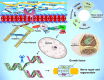
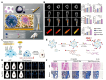
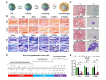
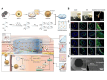
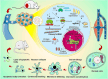
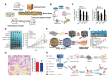
Similar articles
-
Biomaterial-guided delivery of gene vectors for targeted articular cartilage repair.Nat Rev Rheumatol. 2019 Jan;15(1):18-29. doi: 10.1038/s41584-018-0125-2. Nat Rev Rheumatol. 2019. PMID: 30514957 Review.
-
Recent advances in polymeric biomaterials-based gene delivery for cartilage repair.Bioact Mater. 2020 Jul 3;5(4):990-1003. doi: 10.1016/j.bioactmat.2020.06.004. eCollection 2020 Dec. Bioact Mater. 2020. PMID: 32671293 Free PMC article.
-
Bioinspired Star-Shaped Poly(l-lysine) Polypeptides: Efficient Polymeric Nanocarriers for the Delivery of DNA to Mesenchymal Stem Cells.Mol Pharm. 2018 May 7;15(5):1878-1891. doi: 10.1021/acs.molpharmaceut.8b00044. Epub 2018 Apr 6. Mol Pharm. 2018. PMID: 29590755
-
Bone Regeneration Using Gene-Activated Matrices.AAPS J. 2017 Jan;19(1):43-53. doi: 10.1208/s12248-016-9982-2. Epub 2016 Sep 21. AAPS J. 2017. PMID: 27655418 Free PMC article. Review.
-
Constructing Gene-Enhanced Tissue Engineering for Regeneration and Repair of Osteochondral Defects.Adv Biosyst. 2019 Aug;3(8):e1900004. doi: 10.1002/adbi.201900004. Epub 2019 Jun 27. Adv Biosyst. 2019. PMID: 32648702
Cited by
-
Microenvironment-responsive nanomedicines: a promising direction for tissue regeneration.Mil Med Res. 2024 Oct 21;11(1):69. doi: 10.1186/s40779-024-00573-0. Mil Med Res. 2024. PMID: 39434177 Free PMC article. Review.
-
Force-sensing protein expression in response to cardiovascular mechanotransduction.EBioMedicine. 2024 Dec;110:105412. doi: 10.1016/j.ebiom.2024.105412. Epub 2024 Oct 30. EBioMedicine. 2024. PMID: 39481337 Free PMC article. Review.
References
Publication types
MeSH terms
Substances
Grants and funding
LinkOut - more resources
Full Text Sources
Medical