Neuroimmune recognition and regulation in the respiratory system
- PMID: 38925790
- PMCID: PMC11216688
- DOI: 10.1183/16000617.0008-2024
Neuroimmune recognition and regulation in the respiratory system
Abstract
Neuroimmune recognition and regulation in the respiratory system is a complex and highly coordinated process involving interactions between the nervous and immune systems to detect and respond to pathogens, pollutants and other potential hazards in the respiratory tract. This interaction helps maintain the health and integrity of the respiratory system. Therefore, understanding the complex interactions between the respiratory nervous system and immune system is critical to maintaining lung health and developing treatments for respiratory diseases. In this review, we summarise the projection distribution of different types of neurons (trigeminal nerve, glossopharyngeal nerve, vagus nerve, spinal dorsal root nerve, sympathetic nerve) in the respiratory tract. We also introduce several types of cells in the respiratory epithelium that closely interact with nerves (pulmonary neuroendocrine cells, brush cells, solitary chemosensory cells and tastebuds). These cells are primarily located at key positions in the respiratory tract, where nerves project to them, forming neuroepithelial recognition units, thus enhancing the ability of neural recognition. Furthermore, we summarise the roles played by these different neurons in sensing or responding to specific pathogens (influenza, severe acute respiratory syndrome coronavirus 2, respiratory syncytial virus, human metapneumovirus, herpes viruses, Sendai parainfluenza virus, Mycobacterium tuberculosis, Pseudomonas aeruginosa, Staphylococcus aureus, amoebae), allergens, atmospheric pollutants (smoking, exhaust pollution), and their potential roles in regulating interactions among different pathogens. We also summarise the prospects of bioelectronic medicine as a third therapeutic approach following drugs and surgery, as well as the potential mechanisms of meditation breathing as an adjunct therapy.
Copyright ©The authors 2024.
Conflict of interest statement
Conflict of interest: All authors have nothing to disclose.
Figures
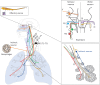
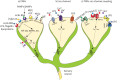
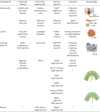
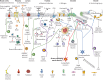
Similar articles
-
Neural Immune Communication in the Control of Host-Bacterial Pathogen Interactions in the Gastrointestinal Tract.Infect Immun. 2020 Aug 19;88(9):e00928-19. doi: 10.1128/IAI.00928-19. Print 2020 Aug 19. Infect Immun. 2020. PMID: 32341116 Free PMC article. Review.
-
Antibody responses after Sendai virus infection and their role in upper and lower respiratory tract disease in rats.Lab Anim Sci. 1999 Aug;49(4):385-94. Lab Anim Sci. 1999. PMID: 10480643
-
Primary afferent projections from the upper respiratory tract in the muskrat.J Comp Neurol. 1991 Jun 1;308(1):51-65. doi: 10.1002/cne.903080106. J Comp Neurol. 1991. PMID: 1714922
-
Respiratory syncytial virus-Host interaction in the pathogenesis of bronchiolitis and its impact on respiratory morbidity in later life.Pediatr Allergy Immunol. 2017 Jun;28(4):320-331. doi: 10.1111/pai.12716. Epub 2017 Apr 26. Pediatr Allergy Immunol. 2017. PMID: 28339145 Review.
-
Vagus nerve cholinergic circuitry to the liver and the gastrointestinal tract in the neuroimmune communicatome.Am J Physiol Gastrointest Liver Physiol. 2018 Nov 1;315(5):G651-G658. doi: 10.1152/ajpgi.00195.2018. Epub 2018 Jul 12. Am J Physiol Gastrointest Liver Physiol. 2018. PMID: 30001146 Free PMC article. Review.
References
Publication types
MeSH terms
LinkOut - more resources
Full Text Sources