Towards Stem Cell Therapy for Critical-Sized Segmental Bone Defects: Current Trends and Challenges on the Path to Clinical Translation
- PMID: 38921519
- PMCID: PMC11205181
- DOI: 10.3390/jfb15060145
Towards Stem Cell Therapy for Critical-Sized Segmental Bone Defects: Current Trends and Challenges on the Path to Clinical Translation
Abstract
The management and reconstruction of critical-sized segmental bone defects remain a major clinical challenge for orthopaedic clinicians and surgeons. In particular, regenerative medicine approaches that involve incorporating stem cells within tissue engineering scaffolds have great promise for fracture management. This narrative review focuses on the primary components of bone tissue engineering-stem cells, scaffolds, the microenvironment, and vascularisation-addressing current advances and translational and regulatory challenges in the current landscape of stem cell therapy for critical-sized bone defects. To comprehensively explore this research area and offer insights for future treatment options in orthopaedic surgery, we have examined the latest developments and advancements in bone tissue engineering, focusing on those of clinical relevance in recent years. Finally, we present a forward-looking perspective on using stem cells in bone tissue engineering for critical-sized segmental bone defects.
Keywords: bone tissue engineering; clinical translation; critical-sized bone defects; mesenchymal stem cells; microenvironment; regulatory framework; vascularisation.
Conflict of interest statement
Y.C. is the founder and shareholder of Plasticell Ltd., United Kingdom. S.H.T. is a co-founder of Osteopore International Pte Ltd., Singapore.
Figures
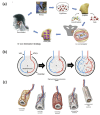
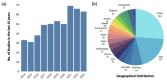
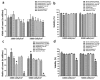
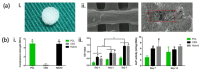
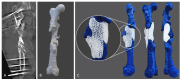
Similar articles
-
Clinical Applications of Bone Tissue Engineering in Orthopedic Trauma.Curr Pathobiol Rep. 2018 Jun;6(2):99-108. doi: 10.1007/s40139-018-0166-x. Epub 2018 Apr 19. Curr Pathobiol Rep. 2018. PMID: 36506709 Free PMC article.
-
Finite element method (FEM), mechanobiology and biomimetic scaffolds in bone tissue engineering.Int J Biol Sci. 2011 Jan 26;7(1):112-32. doi: 10.7150/ijbs.7.112. Int J Biol Sci. 2011. PMID: 21278921 Free PMC article. Review.
-
Structure-optimized and microenvironment-inspired nanocomposite biomaterials in bone tissue engineering.Burns Trauma. 2024 Jun 9;12:tkae036. doi: 10.1093/burnst/tkae036. eCollection 2024. Burns Trauma. 2024. PMID: 38855573 Free PMC article. Review.
-
Mesenchymal stem cells seeded onto tissue-engineered osteoinductive scaffolds enhance the healing process of critical-sized radial bone defects in rat.Cell Tissue Res. 2018 Oct;374(1):63-81. doi: 10.1007/s00441-018-2837-7. Epub 2018 May 1. Cell Tissue Res. 2018. Retraction in: Cell Tissue Res. 2021 Oct;386(1):211. doi: 10.1007/s00441-021-03520-w. PMID: 29717356 Retracted.
-
Treatment of osteochondral defects in the rabbit's knee joint by implantation of allogeneic mesenchymal stem cells in fibrin clots.J Vis Exp. 2013 May 21;(75):e4423. doi: 10.3791/4423. J Vis Exp. 2013. PMID: 23728213 Free PMC article.
Cited by
-
Application of gelatin-based composites in bone tissue engineering.Heliyon. 2024 Aug 14;10(16):e36258. doi: 10.1016/j.heliyon.2024.e36258. eCollection 2024 Aug 30. Heliyon. 2024. PMID: 39224337 Free PMC article. Review.
References
Publication types
Grants and funding
LinkOut - more resources
Full Text Sources