This is a preprint.
Synthetic genomes unveil the effects of synonymous recoding
- PMID: 38915524
- PMCID: PMC11195188
- DOI: 10.1101/2024.06.16.599206
Synthetic genomes unveil the effects of synonymous recoding
Abstract
Engineering the genetic code of an organism provides the basis for (i) making any organism safely resistant to natural viruses and (ii) preventing genetic information flow into and out of genetically modified organisms while (iii) allowing the biosynthesis of genetically encoded unnatural polymers1-4. Achieving these three goals requires the reassignment of multiple of the 64 codons nature uses to encode proteins. However, synonymous codon replacement-recoding-is frequently lethal, and how recoding impacts fitness remains poorly explored. Here, we explore these effects using whole-genome synthesis, multiplexed directed evolution, and genome-transcriptome-translatome-proteome co-profiling on multiple recoded genomes. Using this information, we assemble a synthetic Escherichia coli genome in seven sections using only 57 codons to encode proteins. By discovering the rules responsible for the lethality of synonymous recoding and developing a data-driven multi-omics-based genome construction workflow that troubleshoots synthetic genomes, we overcome the lethal effects of 62,007 synonymous codon swaps and 11,108 additional genomic edits. We show that synonymous recoding induces transcriptional noise including new antisense RNAs, leading to drastic transcriptome and proteome perturbation. As the elimination of select codons from an organism's genetic code results in the widespread appearance of cryptic promoters, we show that synonymous codon choice may naturally evolve to minimize transcriptional noise. Our work provides the first genome-scale description of how synonymous codon changes influence organismal fitness and paves the way for the construction of functional genomes that provide genetic firewalls from natural ecosystems and safely produce biopolymers, drugs, and enzymes with an expanded chemistry.
Conflict of interest statement
Conflict of interest statement The authors declare competing financial interests. A.N. is an inventor on a patent related to directed evolution with random genomic mutations (DIvERGE) (US10669537B2: Mutagenizing Intracellular Nucleic Acids) that has been outlicensed. Harvard Medical School has filed provisional patent applications related to this work on which A.N. and G.M.C. are listed as inventors. Q.Z., M.W., M.L., A.J., K.C., Z.L., and F.H. are employed by GenScript USA Inc., but the company had no role in designing or executing experiments. G.M.C. is a founder of GRO Biosciences and EnEvolv (now part of Ginkgo Bioworks), in which he has related financial interests. Other potentially relevant financial interests of G.M.C. are listed at http://arep.med.harvard.edu/gmc/tech.html.
Figures
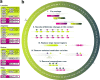
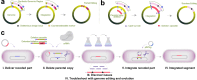
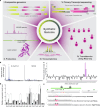
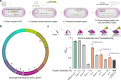
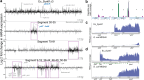
Similar articles
-
Defining synonymous codon compression schemes by genome recoding.Nature. 2016 Nov 3;539(7627):59-64. doi: 10.1038/nature20124. Epub 2016 Oct 24. Nature. 2016. PMID: 27776354 Free PMC article.
-
Attenuation of Human Respiratory Viruses by Synonymous Genome Recoding.Front Immunol. 2019 Jun 4;10:1250. doi: 10.3389/fimmu.2019.01250. eCollection 2019. Front Immunol. 2019. PMID: 31231383 Free PMC article. Review.
-
Total synthesis of Escherichia coli with a recoded genome.Nature. 2019 May;569(7757):514-518. doi: 10.1038/s41586-019-1192-5. Epub 2019 May 15. Nature. 2019. PMID: 31092918 Free PMC article.
-
Design, synthesis, and testing toward a 57-codon genome.Science. 2016 Aug 19;353(6301):819-22. doi: 10.1126/science.aaf3639. Science. 2016. PMID: 27540174
-
Synonymous genome recoding: a tool to explore microbial biology and new therapeutic strategies.Nucleic Acids Res. 2019 Nov 18;47(20):10506-10519. doi: 10.1093/nar/gkz831. Nucleic Acids Res. 2019. PMID: 31584076 Free PMC article. Review.
Cited by
-
The design and engineering of synthetic genomes.Nat Rev Genet. 2024 Nov 6. doi: 10.1038/s41576-024-00786-y. Online ahead of print. Nat Rev Genet. 2024. PMID: 39506144 Review.
References
Publication types
Grants and funding
LinkOut - more resources
Full Text Sources
Research Materials