This is a preprint.
Identification of molecular determinants of gene-specific bursting patterns by high-throughput imaging screens
- PMID: 38903099
- PMCID: PMC11188098
- DOI: 10.1101/2024.06.08.597999
Identification of molecular determinants of gene-specific bursting patterns by high-throughput imaging screens
Abstract
Stochastic transcriptional bursting is a universal property of active genes. While different genes exhibit distinct bursting patterns, the molecular mechanisms for gene-specific stochastic bursting are largely unknown. We have developed and applied a high-throughput-imaging based screening strategy to identify cellular factors and molecular mechanisms that determine the bursting behavior of human genes. Focusing on epigenetic regulators, we find that protein acetylation is a strong acute modulator of burst frequency, burst size and heterogeneity of bursting. Acetylation globally affects the Off-time of genes but has gene-specific effects on the On-time. Yet, these effects are not strongly linked to promoter acetylation, which do not correlate with bursting properties, and forced promoter acetylation has variable effects on bursting. Instead, we demonstrate acetylation of the Integrator complex as a key determinant of gene bursting. Specifically, we find that elevated Integrator acetylation decreases bursting frequency. Taken together our results suggest a prominent role of non-histone proteins in determining gene bursting properties, and they identify histone-independent acetylation of a transcription cofactor as an allosteric modulator of bursting via a far-downstream bursting checkpoint.
Keywords: Integrator complex; Stochastic gene bursting; acetylation; high-throughput imaging screen.
Conflict of interest statement
Declaration of interests The authors declare no competing interests.
Figures
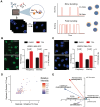
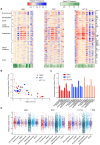
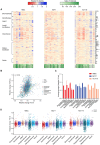
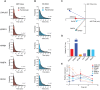
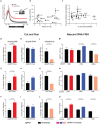
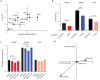
Similar articles
-
Modulation of transcriptional burst frequency by histone acetylation.Proc Natl Acad Sci U S A. 2018 Jul 3;115(27):7153-7158. doi: 10.1073/pnas.1722330115. Epub 2018 Jun 18. Proc Natl Acad Sci U S A. 2018. PMID: 29915087 Free PMC article.
-
Enhancer Histone Acetylation Modulates Transcriptional Bursting Dynamics of Neuronal Activity-Inducible Genes.Cell Rep. 2019 Jan 29;26(5):1174-1188.e5. doi: 10.1016/j.celrep.2019.01.032. Cell Rep. 2019. PMID: 30699347 Free PMC article.
-
What shapes eukaryotic transcriptional bursting?Mol Biosyst. 2017 Jun 27;13(7):1280-1290. doi: 10.1039/c7mb00154a. Mol Biosyst. 2017. PMID: 28573295 Review.
-
Kinetic characteristics of transcriptional bursting in a complex gene model with cyclic promoter structure.Math Biosci Eng. 2022 Jan 24;19(4):3313-3336. doi: 10.3934/mbe.2022153. Math Biosci Eng. 2022. PMID: 35341253
-
Transcriptional bursting dynamics in gene expression.Front Genet. 2024 Sep 13;15:1451461. doi: 10.3389/fgene.2024.1451461. eCollection 2024. Front Genet. 2024. PMID: 39346775 Free PMC article. Review.
References
Publication types
Grants and funding
LinkOut - more resources
Full Text Sources
Research Materials