Before Translating Extracellular Vesicles into Personalized Diagnostics and Therapeutics: What We Could Do
- PMID: 38771015
- PMCID: PMC11151200
- DOI: 10.1021/acs.molpharmaceut.4c00185
Before Translating Extracellular Vesicles into Personalized Diagnostics and Therapeutics: What We Could Do
Abstract
Extracellular vesicle (EV) research is rapidly advancing from fundamental science to translational applications in EV-based personalized therapeutics and diagnostics. Yet, fundamental questions persist regarding EV biology and mechanisms, particularly concerning the heterogeneous interactions between EVs and cells. While we have made strides in understanding virus delivery and intracellular vesicle transport, our comprehension of EV trafficking remains limited. EVs are believed to mediate intercellular communication through cargo transfer, but uncertainties persist regarding the occurrence and quantification of EV-cargo delivery within acceptor cells. This ambiguity is crucial to address, given the significant translational impact of EVs on therapeutics and diagnostics. This perspective article does not seek to provide exhaustive recommendations and guidance on EV-related studies, as these are well-articulated in position papers and statements by the International Society for Extracellular Vesicles (ISEV), including the 'Minimum Information for Studies of Extracellular Vesicles' (MISEV) 2014, MISEV2018, and the recent MISEV2023. Instead, recognizing the multilayered heterogeneity of EVs as both a challenge and an opportunity, this perspective emphasizes novel approaches to facilitate our understanding of diverse EV biology, address uncertainties, and leverage this knowledge to advance EV-based personalized diagnostics and therapeutics. Specifically, this perspective synthesizes current insights, identifies opportunities, and highlights exciting technological advancements in ultrasensitive single EV or "digital" profiling developed within the author's multidisciplinary group. These newly developed technologies address technical gaps in dissecting the molecular contents of EV subsets, contributing to the evolution of EVs as next-generation liquid biopsies for diagnostics and providing better quality control for EV-based therapeutics.
Keywords: diagnostics; drug delivery; exosome; extracellular vesicle; personalized medicine; protein biomarker; single molecule array.
Conflict of interest statement
The author declares no competing financial interest.
Figures
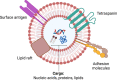
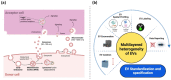
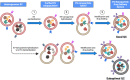
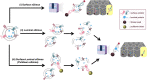
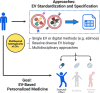
Similar articles
-
Minimal information for studies of extracellular vesicles (MISEV2023): From basic to advanced approaches.J Extracell Vesicles. 2024 Feb;13(2):e12404. doi: 10.1002/jev2.12404. J Extracell Vesicles. 2024. PMID: 38326288 Free PMC article.
-
Comparative analysis of the minimal information for studies of extracellular vesicles guidelines: Advancements and implications for extracellular vesicle research.Semin Cancer Biol. 2024 Jun;101:12-24. doi: 10.1016/j.semcancer.2024.04.002. Epub 2024 Apr 23. Semin Cancer Biol. 2024. PMID: 38657746 Review.
-
Biogenesis, Membrane Trafficking, Functions, and Next Generation Nanotherapeutics Medicine of Extracellular Vesicles.Int J Nanomedicine. 2021 May 18;16:3357-3383. doi: 10.2147/IJN.S310357. eCollection 2021. Int J Nanomedicine. 2021. PMID: 34040369 Free PMC article. Review.
-
"Liquid biopsy" - extracellular vesicles as potential novel players towards precision medicine in asthma.Front Immunol. 2022 Nov 17;13:1025348. doi: 10.3389/fimmu.2022.1025348. eCollection 2022. Front Immunol. 2022. PMID: 36466836 Free PMC article. Review.
-
Characterizing Extracellular Vesicles and Their Diverse RNA Contents.Front Genet. 2020 Jul 17;11:700. doi: 10.3389/fgene.2020.00700. eCollection 2020. Front Genet. 2020. PMID: 32765582 Free PMC article. Review.
Cited by
-
Innovative Strategies to Combat 5-Fluorouracil Resistance in Colorectal Cancer: The Role of Phytochemicals and Extracellular Vesicles.Int J Mol Sci. 2024 Jul 8;25(13):7470. doi: 10.3390/ijms25137470. Int J Mol Sci. 2024. PMID: 39000577 Free PMC article. Review.
References
-
- Welsh J. A.; Goberdhan D. C. I.; O’Driscoll L.; Buzas E. I.; Blenkiron C.; Bussolati B.; Cai H.; Di Vizio D.; Driedonks T. A. P.; Erdbrügger U.; et al. Minimal information for studies of extracellular vesicles (MISEV2023): From basic to advanced approaches. J. Extracell. Vesicles 2024, 13 (2), e12404.10.1002/jev2.12404. - DOI - PMC - PubMed
-
- Pegtel D. M.; Cosmopoulos K.; Thorley-Lawson D. A.; van Eijndhoven M. A.; Hopmans E. S.; Lindenberg J. L.; de Gruijl T. D.; Würdinger T.; Middeldorp J. M. Functional delivery of viral miRNAs via exosomes. Proc. Natl. Acad. Sci. U. S. A. 2010, 107 (14), 6328–6333. 10.1073/pnas.0914843107. - DOI - PMC - PubMed
Publication types
MeSH terms
LinkOut - more resources
Full Text Sources