Microfluidic Biochips for Single-Cell Isolation and Single-Cell Analysis of Multiomics and Exosomes
- PMID: 38767182
- PMCID: PMC11267386
- DOI: 10.1002/advs.202401263
Microfluidic Biochips for Single-Cell Isolation and Single-Cell Analysis of Multiomics and Exosomes
Abstract
Single-cell multiomic and exosome analyses are potent tools in various fields, such as cancer research, immunology, neuroscience, microbiology, and drug development. They facilitate the in-depth exploration of biological systems, providing insights into disease mechanisms and aiding in treatment. Single-cell isolation, which is crucial for single-cell analysis, ensures reliable cell isolation and quality control for further downstream analyses. Microfluidic chips are small lightweight systems that facilitate efficient and high-throughput single-cell isolation and real-time single-cell analysis on- or off-chip. Therefore, most current single-cell isolation and analysis technologies are based on the single-cell microfluidic technology. This review offers comprehensive guidance to researchers across different fields on the selection of appropriate microfluidic chip technologies for single-cell isolation and analysis. This review describes the design principles, separation mechanisms, chip characteristics, and cellular effects of various microfluidic chips available for single-cell isolation. Moreover, this review highlights the implications of using this technology for subsequent analyses, including single-cell multiomic and exosome analyses. Finally, the current challenges and future prospects of microfluidic chip technology are outlined for multiplex single-cell isolation and multiomic and exosome analyses.
Keywords: isolation quality indicators; microfluidic chips; multiomics and exosome applications; single cells.
© 2024 The Authors. Advanced Science published by Wiley‐VCH GmbH.
Conflict of interest statement
The authors declare no conflict of interest.
Figures
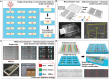
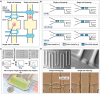
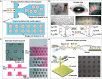
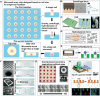
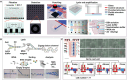
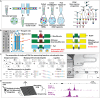
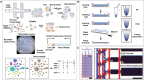
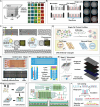
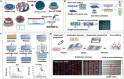
Similar articles
-
Recent advances in microfluidic technologies for separation of biological cells.Biomed Microdevices. 2020 Aug 14;22(3):55. doi: 10.1007/s10544-020-00510-7. Biomed Microdevices. 2020. PMID: 32797312 Review.
-
Flow-electricity coupling fields enhance microfluidic platforms for efficient exosome isolation.Anal Methods. 2024 Aug 1;16(30):5335-5344. doi: 10.1039/d4ay00740a. Anal Methods. 2024. PMID: 39034856
-
[Microfluidic strategies for separation and analysis of circulating exosomes].Se Pu. 2021 Sep;39(9):968-980. doi: 10.3724/SP.J.1123.2021.07005. Se Pu. 2021. PMID: 34486836 Free PMC article. Chinese.
-
Improved Teflon lift-off for droplet microarray generation and single-cell separation on digital microfluidic chips.Lab Chip. 2024 Oct 9;24(20):4869-4878. doi: 10.1039/d4lc00630e. Lab Chip. 2024. PMID: 39301608
-
Microfluidic technologies.Recent Results Cancer Res. 2012;195:59-67. doi: 10.1007/978-3-642-28160-0_5. Recent Results Cancer Res. 2012. PMID: 22527494 Review.
Cited by
-
Extracellular Vesicles: Diagnostic and Therapeutic Applications in Cancer.Biology (Basel). 2024 Sep 12;13(9):716. doi: 10.3390/biology13090716. Biology (Basel). 2024. PMID: 39336143 Free PMC article. Review.
References
Publication types
MeSH terms
Grants and funding
- 2023YFB3210400/National Key R&D Plan of China
- 62174101/National Science Foundation of China
- 32001018/National Science Foundation of China
- 2022JC001/Shandong University Integrated Research and Cultivation Project
- 22-3-4-xxgg-2-nsh/Future industries cultivate project-2022 emerging industries cultivate plan of Qingdao
LinkOut - more resources
Full Text Sources