This is a preprint.
The guide RNA sequence dictates the slicing kinetics and conformational dynamics of the Argonaute silencing complex
- PMID: 38766062
- PMCID: PMC11100590
- DOI: 10.1101/2023.10.15.562437
The guide RNA sequence dictates the slicing kinetics and conformational dynamics of the Argonaute silencing complex
Update in
-
The guide-RNA sequence dictates the slicing kinetics and conformational dynamics of the Argonaute silencing complex.Mol Cell. 2024 Aug 8;84(15):2918-2934.e11. doi: 10.1016/j.molcel.2024.06.026. Epub 2024 Jul 17. Mol Cell. 2024. PMID: 39025072
Abstract
The RNA-induced silencing complex (RISC), which powers RNA interference (RNAi), consists of a guide RNA and an Argonaute protein that slices target RNAs complementary to the guide. We find that for different guide-RNA sequences, slicing rates of perfectly complementary, bound targets can be surprisingly different (>250-fold range), and that faster slicing confers better knockdown in cells. Nucleotide sequence identities at guide-RNA positions 7, 10, and 17 underlie much of this variation in slicing rates. Analysis of one of these determinants implicates a structural distortion at guide nucleotides 6-7 in promoting slicing. Moreover, slicing directed by different guide sequences has an unanticipated, 600-fold range in 3'-mismatch tolerance, attributable to guides with weak (AU-rich) central pairing requiring extensive 3' complementarity (pairing beyond position 16) to more fully populate the slicing-competent conformation. Together, our analyses identify sequence determinants of RISC activity and provide biochemical and conformational rationale for their action.
Keywords: AGO2; Argonaute; RISC; RNAi; RNA–protein interactions; kinetic analysis; microRNA; siRNA; slicing.
Conflict of interest statement
DECLARATION OF INTERESTS D.P.B. has equity in Alnylam Pharmaceuticals, where he is a co-founder and advisor. D.P.B. is a member of Molecular Cell’s advisory board. P.Y.W. declares no competing interests.
Figures
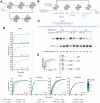
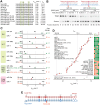
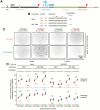
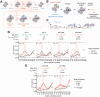
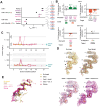
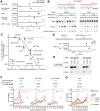
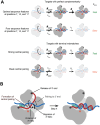
Similar articles
-
The guide-RNA sequence dictates the slicing kinetics and conformational dynamics of the Argonaute silencing complex.Mol Cell. 2024 Aug 8;84(15):2918-2934.e11. doi: 10.1016/j.molcel.2024.06.026. Epub 2024 Jul 17. Mol Cell. 2024. PMID: 39025072
-
Kinetic Analysis of Target RNA Binding and Slicing by Human Argonaute 2 Protein.Methods Mol Biol. 2017;1517:277-290. doi: 10.1007/978-1-4939-6563-2_19. Methods Mol Biol. 2017. PMID: 27924489
-
A Seed Mismatch Enhances Argonaute2-Catalyzed Cleavage and Partially Rescues Severely Impaired Cleavage Found in Fish.Mol Cell. 2017 Dec 21;68(6):1095-1107.e5. doi: 10.1016/j.molcel.2017.11.032. Mol Cell. 2017. PMID: 29272705 Free PMC article.
-
siRNA Specificity: RNAi Mechanisms and Strategies to Reduce Off-Target Effects.Front Plant Sci. 2021 Jan 28;11:526455. doi: 10.3389/fpls.2020.526455. eCollection 2020. Front Plant Sci. 2021. PMID: 33584737 Free PMC article. Review.
-
Life of RISC: Formation, action, and degradation of RNA-induced silencing complex.Mol Cell. 2022 Jan 6;82(1):30-43. doi: 10.1016/j.molcel.2021.11.026. Epub 2021 Dec 22. Mol Cell. 2022. PMID: 34942118 Review.
References
Publication types
Grants and funding
LinkOut - more resources
Full Text Sources