A Review of the Evidence for Tryptophan and the Kynurenine Pathway as a Regulator of Stem Cell Niches in Health and Disease
- PMID: 38757094
- PMCID: PMC11097742
- DOI: 10.1177/11786469241248287
A Review of the Evidence for Tryptophan and the Kynurenine Pathway as a Regulator of Stem Cell Niches in Health and Disease
Abstract
Stem cells are ubiquitously found in various tissues and organs in the body, and underpin the body's ability to repair itself following injury or disease initiation, though repair can sometimes be compromised. Understanding how stem cells are produced, and functional signaling systems between different niches is critical to understanding the potential use of stem cells in regenerative medicine. In this context, this review considers kynurenine pathway (KP) metabolism in multipotent adult progenitor cells, embryonic, haematopoietic, neural, cancer, cardiac and induced pluripotent stem cells, endothelial progenitor cells, and mesenchymal stromal cells. The KP is the major enzymatic pathway for sequentially catabolising the essential amino acid tryptophan (TRP), resulting in key metabolites including kynurenine, kynurenic acid, and quinolinic acid (QUIN). QUIN metabolism transitions into the adjoining de novo pathway for nicotinamide adenine dinucleotide (NAD) production, a critical cofactor in many fundamental cellular biochemical pathways. How stem cells uptake and utilise TRP varies between different species and stem cell types, because of their expression of transporters and responses to inflammatory cytokines. Several KP metabolites are physiologically active, with either beneficial or detrimental outcomes, and evidence of this is presented relating to several stem cell types, which is important as they may exert a significant impact on surrounding differentiated cells, particularly if they metabolise or secrete metabolites differently. Interferon-gamma (IFN-γ) in mesenchymal stromal cells, for instance, highly upregulates rate-limiting enzyme indoleamine-2,3-dioxygenase (IDO-1), initiating TRP depletion and production of metabolites including kynurenine/kynurenic acid, known agonists of the Aryl hydrocarbon receptor (AhR) transcription factor. AhR transcriptionally regulates an immunosuppressive phenotype, making them attractive for regenerative therapy. We also draw attention to important gaps in knowledge for future studies, which will underpin future application for stem cell-based cellular therapies or optimising drugs which can modulate the KP in innate stem cell populations, for disease treatment.
Keywords: IDO-1; TDO2; Tryptophan metabolism; aryl hydrocarbon receptor; cancer stem cells; hematopoietic stem cells; human diseases; induced pluripotent stem cells; kynurenine pathway; mesenchymal stromal cells; neural stem cells.
© The Author(s) 2024.
Conflict of interest statement
The author(s) declared the following potential conflicts of interest with respect to the research, authorship, and/or publication of this article: Prof. Bruce Brew is a member of the International Journal for Tryptophan Research journal editorial board. The other co-authors declare that they have no conflict of interest.
Figures
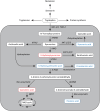
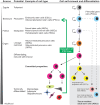
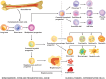
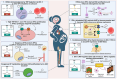
Similar articles
-
Involvement of the kynurenine pathway in human glioma pathophysiology.PLoS One. 2014 Nov 21;9(11):e112945. doi: 10.1371/journal.pone.0112945. eCollection 2014. PLoS One. 2014. PMID: 25415278 Free PMC article.
-
Kynurenine pathway in Parkinson's disease-An update.eNeurologicalSci. 2020 Sep 10;21:100270. doi: 10.1016/j.ensci.2020.100270. eCollection 2020 Dec. eNeurologicalSci. 2020. PMID: 33134567 Free PMC article. Review.
-
Recent evidence for an expanded role of the kynurenine pathway of tryptophan metabolism in neurological diseases.Neuropharmacology. 2017 Jan;112(Pt B):373-388. doi: 10.1016/j.neuropharm.2016.03.024. Epub 2016 Mar 16. Neuropharmacology. 2017. PMID: 26995730 Review.
-
Investigation of the aryl hydrocarbon receptor and the intrinsic tumoral component of the kynurenine pathway of tryptophan metabolism in primary brain tumors.J Neurooncol. 2018 Sep;139(2):239-249. doi: 10.1007/s11060-018-2869-6. Epub 2018 Apr 17. J Neurooncol. 2018. PMID: 29667084 Free PMC article.
-
The kynurenine pathway in brain tumor pathogenesis.Cancer Res. 2012 Nov 15;72(22):5649-57. doi: 10.1158/0008-5472.CAN-12-0549. Epub 2012 Nov 9. Cancer Res. 2012. PMID: 23144293 Review.
Cited by
-
The Complex World of Kynurenic Acid: Reflections on Biological Issues and Therapeutic Strategy.Int J Mol Sci. 2024 Aug 20;25(16):9040. doi: 10.3390/ijms25169040. Int J Mol Sci. 2024. PMID: 39201726 Free PMC article. Review.
References
Publication types
LinkOut - more resources
Full Text Sources
Research Materials