Biosensor-Enhanced Organ-on-a-Chip Models for Investigating Glioblastoma Tumor Microenvironment Dynamics
- PMID: 38732975
- PMCID: PMC11086276
- DOI: 10.3390/s24092865
Biosensor-Enhanced Organ-on-a-Chip Models for Investigating Glioblastoma Tumor Microenvironment Dynamics
Abstract
Glioblastoma, an aggressive primary brain tumor, poses a significant challenge owing to its dynamic and intricate tumor microenvironment. This review investigates the innovative integration of biosensor-enhanced organ-on-a-chip (OOC) models as a novel strategy for an in-depth exploration of glioblastoma tumor microenvironment dynamics. In recent years, the transformative approach of incorporating biosensors into OOC platforms has enabled real-time monitoring and analysis of cellular behaviors within a controlled microenvironment. Conventional in vitro and in vivo models exhibit inherent limitations in accurately replicating the complex nature of glioblastoma progression. This review addresses the existing research gap by pioneering the integration of biosensor-enhanced OOC models, providing a comprehensive platform for investigating glioblastoma tumor microenvironment dynamics. The applications of this combined approach in studying glioblastoma dynamics are critically scrutinized, emphasizing its potential to bridge the gap between simplistic models and the intricate in vivo conditions. Furthermore, the article discusses the implications of biosensor-enhanced OOC models in elucidating the dynamic features of the tumor microenvironment, encompassing cell migration, proliferation, and interactions. By furnishing real-time insights, these models significantly contribute to unraveling the complex biology of glioblastoma, thereby influencing the development of more accurate diagnostic and therapeutic strategies.
Keywords: biosensor; cellular dynamics; diagnostic strategies; glioblastoma; microenvironment; organ-on-a-chip; precision medicine; real-time monitoring; therapeutic approaches.
Conflict of interest statement
The authors declare no conflicts of interest.
Figures
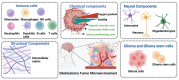
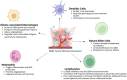
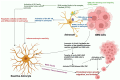
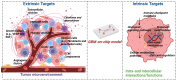
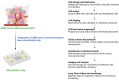
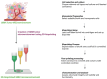
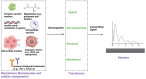
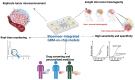
Similar articles
-
A Comprehensive Review of Organ-on-a-Chip Technology and Its Applications.Biosensors (Basel). 2024 May 1;14(5):225. doi: 10.3390/bios14050225. Biosensors (Basel). 2024. PMID: 38785699 Free PMC article. Review.
-
Biosensors integrated 3D organoid/organ-on-a-chip system: A real-time biomechanical, biophysical, and biochemical monitoring and characterization.Biosens Bioelectron. 2023 Jul 1;231:115285. doi: 10.1016/j.bios.2023.115285. Epub 2023 Apr 7. Biosens Bioelectron. 2023. PMID: 37058958 Review.
-
Revolutionizing Drug Discovery: The Impact of Distinct Designs and Biosensor Integration in Microfluidics-Based Organ-on-a-Chip Technology.Biosensors (Basel). 2024 Sep 3;14(9):425. doi: 10.3390/bios14090425. Biosensors (Basel). 2024. PMID: 39329800 Free PMC article. Review.
-
Organ-On-A-Chip: An Emerging Research Platform.Organogenesis. 2023 Dec 31;19(1):2278236. doi: 10.1080/15476278.2023.2278236. Epub 2023 Nov 15. Organogenesis. 2023. PMID: 37965897 Free PMC article. Review.
-
Organ-on-Chip platforms to study tumor evolution and chemosensitivity.Biochim Biophys Acta Rev Cancer. 2022 May;1877(3):188717. doi: 10.1016/j.bbcan.2022.188717. Epub 2022 Mar 16. Biochim Biophys Acta Rev Cancer. 2022. PMID: 35304293 Review.
References
Publication types
MeSH terms
Grants and funding
LinkOut - more resources
Full Text Sources
Miscellaneous