Bruno 1/CELF regulates splicing and cytoskeleton dynamics to ensure correct sarcomere assembly in Drosophila flight muscles
- PMID: 38683844
- PMCID: PMC11081514
- DOI: 10.1371/journal.pbio.3002575
Bruno 1/CELF regulates splicing and cytoskeleton dynamics to ensure correct sarcomere assembly in Drosophila flight muscles
Abstract
Muscles undergo developmental transitions in gene expression and alternative splicing that are necessary to refine sarcomere structure and contractility. CUG-BP and ETR-3-like (CELF) family RNA-binding proteins are important regulators of RNA processing during myogenesis that are misregulated in diseases such as Myotonic Dystrophy Type I (DM1). Here, we report a conserved function for Bruno 1 (Bru1, Arrest), a CELF1/2 family homolog in Drosophila, during early muscle myogenesis. Loss of Bru1 in flight muscles results in disorganization of the actin cytoskeleton leading to aberrant myofiber compaction and defects in pre-myofibril formation. Temporally restricted rescue and RNAi knockdown demonstrate that early cytoskeletal defects interfere with subsequent steps in sarcomere growth and maturation. Early defects are distinct from a later requirement for bru1 to regulate sarcomere assembly dynamics during myofiber maturation. We identify an imbalance in growth in sarcomere length and width during later stages of development as the mechanism driving abnormal radial growth, myofibril fusion, and the formation of hollow myofibrils in bru1 mutant muscle. Molecularly, we characterize a genome-wide transition from immature to mature sarcomere gene isoform expression in flight muscle development that is blocked in bru1 mutants. We further demonstrate that temporally restricted Bru1 rescue can partially alleviate hypercontraction in late pupal and adult stages, but it cannot restore myofiber function or correct structural deficits. Our results reveal the conserved nature of CELF function in regulating cytoskeletal dynamics in muscle development and demonstrate that defective RNA processing due to misexpression of CELF proteins causes wide-reaching structural defects and progressive malfunction of affected muscles that cannot be rescued by late-stage gene replacement.
Copyright: © 2024 Nikonova et al. This is an open access article distributed under the terms of the Creative Commons Attribution License, which permits unrestricted use, distribution, and reproduction in any medium, provided the original author and source are credited.
Conflict of interest statement
The authors have declared that no competing interests exist.
Figures
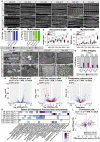
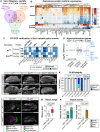
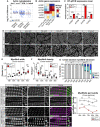
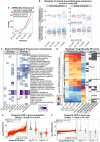
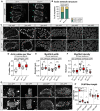
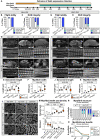
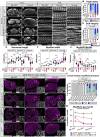
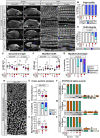
Similar articles
-
The RNA-binding protein Arrest (Bruno) regulates alternative splicing to enable myofibril maturation in Drosophila flight muscle.EMBO Rep. 2015 Feb;16(2):178-91. doi: 10.15252/embr.201439791. Epub 2014 Dec 22. EMBO Rep. 2015. PMID: 25532219 Free PMC article.
-
A Candidate RNAi Screen Reveals Diverse RNA-Binding Protein Phenotypes in Drosophila Flight Muscle.Cells. 2021 Sep 22;10(10):2505. doi: 10.3390/cells10102505. Cells. 2021. PMID: 34685485 Free PMC article.
-
Bruno-3 regulates sarcomere component expression and contributes to muscle phenotypes of myotonic dystrophy type 1.Dis Model Mech. 2018 May 21;11(5):dmm031849. doi: 10.1242/dmm.031849. Dis Model Mech. 2018. PMID: 29716962 Free PMC article.
-
The Muscleblind family of proteins: an emerging class of regulators of developmentally programmed alternative splicing.Differentiation. 2006 Mar;74(2-3):65-80. doi: 10.1111/j.1432-0436.2006.00060.x. Differentiation. 2006. PMID: 16533306 Review.
-
Indirect flight muscles in Drosophila melanogaster as a tractable model to study muscle development and disease.Int J Dev Biol. 2020;64(1-2-3):167-173. doi: 10.1387/ijdb.190333un. Int J Dev Biol. 2020. PMID: 32659005 Review.
References
Publication types
MeSH terms
Substances
Grants and funding
LinkOut - more resources
Full Text Sources
Molecular Biology Databases